3020 Lecture 8
Amber Stokes・2 minutes read
The nervous system relies on membrane potential for its performance, with neurons able to alter this potential through shifting charges across the cell's surface. Understanding the movement of ions, changes in permeability, and the distinct stages of action potential is crucial for comprehending the complex process and preparing for exam questions.
Insights
- Neurons maintain a resting membrane potential of around -70 millivolts, achieved through the sodium-potassium pump moving ions and potassium leak channels allowing constant diffusion, crucial for generating graded potentials leading to action potentials.
- Action potentials follow a precise sequence involving depolarization, repolarization, and hyperpolarization stages, driven by the opening and closing of voltage-gated sodium and potassium channels, emphasizing the importance of understanding ion movements and channel states for a comprehensive grasp of neural signaling processes.
Get key ideas from YouTube videos. It’s free
Recent questions
What is membrane potential?
The difference in electric potential between cell interior and exterior.
How do neurons generate action potentials?
By reaching a certain threshold and depolarizing.
What are EPSPs and IPSPs?
Excitatory and inhibitory post-synaptic potentials.
What is the role of voltage-gated channels?
Crucial in action potentials for ion movement.
Why is understanding ion movement important?
Essential for comprehending action potential processes.
Related videos
Summary
00:00
"Neurons and Membrane Potential: A Summary"
- The nervous system's performance relies on membrane potential, a difference in electric potential between a cell's interior and exterior.
- Neurons can alter this membrane potential, unlike other cells, by shifting charges across the cell's surface.
- Cells maintain a negative charge inside and a positive charge outside, with high potassium and anion concentrations inside and high sodium and chloride concentrations outside.
- Neurons have membrane potential fluctuations due to temporary disruptions, distinct from other cells.
- Resting membrane potential is around -70 millivolts, maintained by the sodium-potassium pump and potassium leak channels.
- The sodium-potassium pump moves two potassium ions in and three sodium ions out, resulting in a net negative charge inside the cell.
- Potassium leak channels allow potassium to constantly diffuse out, contributing to the net negative charge inside the cell.
- Graded potentials, varying in size, can be excitatory (depolarizations) or inhibitory (hyperpolarizations), adding their effects in the cell body.
- If graded potentials reach threshold, an action potential is generated for long-distance transmission down an axon.
- Excitatory post-synaptic potentials (EPSPs) involve neurotransmitters binding to ligand-gated channels, allowing sodium influx and depolarization, while inhibitory post-synaptic potentials (IPSPs) cause hyperpolarization by chloride influx.
29:54
"Cell Signals Trigger Action Potential Process"
- Signals in a cell must be strong enough to trigger an action potential, which occurs when they reach a certain threshold, typically around -55 millivolts.
- For signals to result in a response, they need to be close together, as distant signals may not be effective in generating an action potential.
- Negative signals must also be considered and added to the positive signals to determine if the threshold for an action potential is met.
- Action potentials are all the same size and are not additive, following an "all or none" principle where they either occur if the threshold is met or do not happen if it isn't.
- The process of an action potential involves stages of depolarization, repolarization, and hyperpolarization, leading to the cell returning to its resting state.
- Voltage-gated sodium channels play a crucial role in action potentials, with an activation gate opening at -55 millivolts and an inactivation gate preventing reopening until the resting membrane potential is reached.
- Voltage-gated potassium channels, unlike sodium channels, have only one gate and open or close in response to membrane potential changes.
- During an action potential, sodium rushes into the cell during depolarization, while potassium leaks out and then rushes out during repolarization.
- The permeability of sodium and potassium shifts during an action potential, with sodium permeability increasing during depolarization and potassium permeability increasing during repolarization.
- The recovery phase of an action potential involves potassium moving back into the cell through leak channels, causing a temporary dip below resting membrane potential before equilibrium is reached.
54:44
Understanding Action Potential Process in Neurons
- At rest, the membrane potential is -70 millivolts, with potassium leak channels open and the activation gate of the voltage-gated sodium channel closed while the inactivation gate is open. The voltage-gated potassium channel is closed, with potassium moving out of the cell due to higher permeability compared to sodium.
- During the rising phase, depolarization occurs as the membrane potential rises from -70 to 55 millivolts, heading towards a peak of +40 millivolts. Both the potassium leak channel and the voltage-gated sodium channel are open, with potassium leaking out and sodium rushing in rapidly, leading to a peak in membrane potential.
- The falling phase, or repolarization, sees the membrane potential returning towards -70 millivolts from +40 millivolts. The inactivation gate of the voltage-gated sodium channel closes at +40 millivolts, while the activation gate remains open, and the voltage-gated potassium channel opens, causing potassium to rush out of the cell due to higher permeability than sodium.
- The recovery phase involves a slight hyperpolarization, with the membrane potential dropping to around -90 millivolts before returning to -70 millivolts. The inactivation gate of the voltage-gated sodium channel opens, the activation gate closes, and the voltage-gated potassium channel slowly closes, allowing potassium to continue moving out until the membrane potential stabilizes back to rest.
- It is crucial to be specific in differentiating between potassium leak channels and voltage-gated potassium channels, as well as between activation and inactivation gates for sodium and potassium channels. Understanding the movement of ions and changes in permeability is essential in comprehending the action potential process.
- Repetitive review and practice are recommended to grasp the complex steps involved in the action potential process, as exam questions typically focus on detailing membrane potential changes, channel states, ion movements, and permeability variations. Seeking clarification through office hours or online discussions can aid in solidifying understanding for exams.
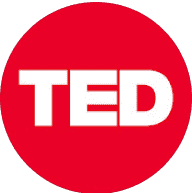
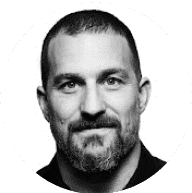
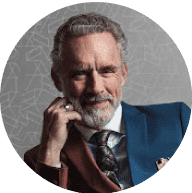

