The Nervous System, Part 2 - Action! Potential!: Crash Course Anatomy & Physiology #9
CrashCourse・2 minutes read
Neurons communicate through action potentials, an electrical signal triggered by a sufficient stimulus that varies in frequency but not strength, while the sodium-potassium pump maintains the resting membrane potential necessary for this signaling. The speed of these signals is enhanced by the myelin sheath, allowing for more efficient conduction, and the importance of this educational content is highlighted for younger audiences through the upcoming Crash Course Kids series.
Insights
- Neurons communicate using a single type of electrical signal known as action potential, which is generated when they are sufficiently stimulated; this signal maintains a consistent strength but varies in frequency, meaning that a stronger stimulus leads to more frequent action potentials, directly impacting muscle contraction intensity.
- The process of action potential generation and propagation relies on the careful balance of ion concentrations maintained by the sodium-potassium pump and the opening of ion channels, with myelinated axons conducting signals more rapidly through saltatory conduction, highlighting the importance of these mechanisms in the overall efficiency of neural communication.
Get key ideas from YouTube videos. It’s free
Recent questions
What is an action potential?
An action potential is a rapid electrical signal that neurons use to communicate. It occurs when a neuron is sufficiently stimulated, causing a change in its membrane potential. This change is initiated when the membrane potential reaches a threshold of approximately -55 millivolts, leading to the opening of voltage-gated sodium channels. Sodium ions rush into the neuron, causing depolarization, which can peak at around +40 millivolts. The action potential is characterized by its all-or-nothing nature, meaning it either occurs fully or not at all, and it varies in frequency based on the strength of the stimulus, affecting how signals are transmitted in the nervous system.
How do neurons maintain resting potential?
Neurons maintain a resting membrane potential of about -70 millivolts through a combination of ion distribution and active transport mechanisms. The sodium-potassium pump plays a crucial role in this process by actively transporting three sodium ions out of the neuron for every two potassium ions it brings in. This creates a polarized state, with a higher concentration of sodium ions outside the neuron and potassium ions inside. The differential distribution of these ions, along with the selective permeability of the neuron's membrane to certain ions, helps establish and maintain the resting potential, which is essential for the proper functioning of neurons and their ability to generate action potentials when stimulated.
What is the role of ion channels?
Ion channels are integral membrane proteins that facilitate the movement of ions in and out of neurons, playing a vital role in the generation and propagation of action potentials. These channels can be voltage-gated, meaning they open or close in response to changes in membrane potential. For instance, when the membrane potential reaches the threshold of -55 millivolts, voltage-gated sodium channels open, allowing sodium ions to flow into the neuron, leading to depolarization. Similarly, potassium channels open during the repolarization phase, allowing potassium ions to exit the neuron. The precise timing and functioning of these ion channels are crucial for the rapid transmission of electrical signals along the axon and for the overall excitability of the neuron.
What is saltatory conduction?
Saltatory conduction is a process that enhances the speed of action potential propagation along myelinated axons. In this mechanism, the action potential does not travel continuously along the entire length of the axon; instead, it "jumps" between the Nodes of Ranvier, which are small gaps in the myelin sheath. This jumping occurs because the myelin insulates the axon, preventing ion flow except at these nodes. As a result, the action potential can travel much faster compared to unmyelinated axons, where the signal must propagate continuously. This efficient conduction mechanism is essential for rapid communication within the nervous system, allowing for quick reflexes and coordinated muscle movements.
What happens during the refractory period?
The refractory period is a critical phase that occurs after an action potential, during which a neuron is temporarily unable to respond to new stimuli. This period ensures that action potentials travel in one direction along the axon and prevents the neuron from being overstimulated. During the absolute refractory period, which follows the depolarization and repolarization phases, the voltage-gated sodium channels are inactivated, and no new action potential can be generated, regardless of the strength of the incoming stimulus. Following this, the relative refractory period occurs, where a stronger-than-normal stimulus is required to initiate another action potential. This mechanism is vital for maintaining the integrity and timing of neuronal signaling, allowing for organized and efficient communication within the nervous system.
Related videos
Summary
00:00
Neurons and Action Potentials Explained
- Neurons communicate impulses through a single type of electrical signal called action potential, which is triggered when a neuron is sufficiently stimulated, resulting in a uniform signal that varies only in frequency, not strength.
- The body operates like a battery, maintaining a resting membrane potential of approximately -70 millivolts, with sodium ions concentrated outside the neuron and potassium ions inside, creating a polarized state.
- The sodium-potassium pump is crucial for maintaining this charge difference, pumping out three sodium ions for every two potassium ions it brings in, thus creating an electrochemical gradient that the body seeks to balance.
- Ion channels in the neuron's membrane allow ions to flow in and out, with voltage-gated channels opening at around -55 millivolts, which is the threshold needed to trigger an action potential.
- When a stimulus causes the membrane potential to reach -55 mV, voltage-gated sodium channels open, allowing sodium ions to rush in and depolarize the neuron to about +40 mV, initiating the action potential.
- Following depolarization, potassium channels open to allow potassium ions to exit the neuron, leading to repolarization and potentially hyperpolarization, where the membrane voltage temporarily drops to around -75 mV.
- The refractory period occurs after an action potential, during which the neuron cannot respond to new stimuli, ensuring that signals travel in one direction along the axon.
- Action potentials are consistent in strength but vary in frequency; a weak stimulus results in fewer action potentials, while a strong stimulus increases the frequency, affecting muscle contraction intensity.
- The speed of action potential conduction is influenced by the presence of a myelin sheath on the axon, with myelinated axons conducting impulses faster through a process called saltatory conduction, where the impulse jumps between Nodes of Ranvier.
- The episode concludes with a mention of the upcoming Crash Course Kids series, which will focus on fifth-grade science topics, ensuring educational content is accessible to younger audiences.
11:22
Subscribe for Engaging Educational Content for Kids
- Teachers, parents, and anyone familiar with children can visit youtube.com/CrashCourseKids to subscribe for educational content.
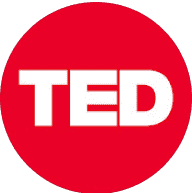
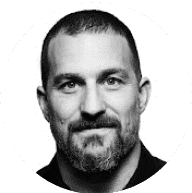
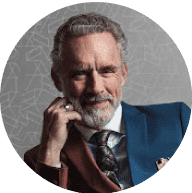

