U4.2: From Gene to Protein
Wolfe Biology Lectures - ARC・102 minutes read
The lecture covers transcription, translation, and mutations in molecular biology, exploring the process of DNA coding for proteins, with key figures like George Beadle and Edward Tatum. It delves into the central dogma of biology, RNA processes, genetic codes deciphered by Nirenberg, and translation mechanisms, emphasizing protein synthesis and genetic engineering through the universal genetic code.
Insights
- George Beadle and Edward Tatum's experiments with Neurospora crassa led to the one gene-one enzyme hypothesis, showing how genes control enzyme production.
- Transcription involves copying DNA into RNA, while translation transforms RNA into proteins, following the central dogma of biology.
- The discovery of the genetic code by Marshall Nirenberg and Heinrich Matine unveiled a universal language shared by all living organisms, enabling genetic engineering across species.
Get key ideas from YouTube videos. It’s free
Recent questions
What is transcription in molecular biology?
Transcription is the process of copying DNA into RNA.
How does translation differ from transcription?
Translation converts RNA into proteins.
What is the one gene-one enzyme hypothesis?
Genes control enzyme production.
What is the central dogma of biology?
Information flows from DNA to RNA to proteins.
Related videos
Parth Momaya
Heredity and Evolution | Lecture 1 - Transcription, Translation and Translocation | MH State Board
Parth Momaya
Heredity and Evolution | Lecture 0 - Basic Concepts | Maharashtra State Board
MIT OpenCourseWare
7. Replication
MIT OpenCourseWare
6. Nucleic Acids
Etoos Education
MOLECULAR BASIS OF INHERITANCE in 1 Shot - All Concepts, Tricks & PYQ's Covered | NEET | ETOOS India
Summary
00:00
"DNA to Proteins: Transcription, Translation, Mutation"
- Lecture focuses on transcription, translation, and mutation in molecular biology, transitioning from DNA discovery to its functionality in coding for proteins.
- Main topics covered are transcription and translation, detailing the process of making RNA from DNA and proteins from RNA.
- George Beadle and Edward Tatum's experiments with Neurospora crassa revealed the one gene-one enzyme hypothesis, showing how genes control enzyme production.
- Beetle and Tatum's research involved irradiating mold with x-rays, leading to mutations affecting the mold's ability to produce essential nutrients.
- Mutations in the mold's genes disrupted the arginine biosynthesis pathway, highlighting the importance of specific enzymes in biological processes.
- Different mutations in the mold's genes affected various steps in the arginine biosynthesis pathway, demonstrating the one gene-one enzyme concept.
- Transcription involves copying DNA into RNA, while translation changes the language from nucleic acids to amino acids to produce proteins.
- The central dogma of biology states that information flows from DNA to RNA to proteins, with exceptions like retroviruses using reverse transcriptase.
- The process of transcription includes adding a cap and tail to mRNA, removing non-coding segments, and processing the RNA before it leaves the nucleus.
- Translation involves TRNA carrying specific amino acids to the ribosome, where they form covalent bonds to create proteins that are then processed and secreted from the cell.
18:42
Decoding RNA: Building Blocks of Life
- RNA is built Phi Prime to three prime, similar to DNA polymerase.
- The template strand is determined by the direction of RNA synthesis.
- Base pairing rules change in RNA transcription due to the absence of thymine, replaced by uracil.
- A common mistake is substituting uracil for thymine directly, but it's a base pairing process.
- Different types of RNA are involved in protein synthesis, with messenger RNA, ribosomal RNA, and transfer RNA being crucial.
- Marshall Nirenberg and Heinrich Matine's experiments in 1961 decoded the genetic code using RNA triplets.
- RNA contains the instructions inherited from parents for protein synthesis.
- Nirenberg deciphered the 64 triplets forming the genetic code, earning a Nobel Prize.
- The genetic code's discovery revealed a universal language shared by all living organisms.
- The genetic code's structure was unraveled through experiments on codons, frame shifts, and redundancy, leading to the understanding of how DNA translates into amino acids.
35:40
Universal genetic code enables cross-species gene expression.
- Methionine and tryptophan have unique codes with no redundancy, unlike other amino acids with multiple codes.
- The genetic code table is universal across all living organisms, including mitochondria and chloroplast genes.
- Genetic engineering benefits from the universal genetic code, allowing genes from one organism to function in another.
- Transgenic pigs expressing jellyfish genes demonstrate the cross-species functionality of genes.
- Genetic engineering in E. coli shows that eukaryotic genes can be expressed in prokaryotes.
- Proteins like human growth hormone and insulin can be produced in bacteria using the universal genetic code.
- Transcription begins with RNA polymerase recognizing and binding to the promoter region on DNA.
- RNA polymerase moves along the DNA template, synthesizing a complementary single-stranded mRNA molecule.
- Transcription termination involves the formation of a stem-loop structure by the RNA, halting RNA polymerase movement.
- The initiation and elongation of transcription are precise processes guided by specific promoter sequences and terminator sites.
54:55
Platypus DNA, Prokaryotes, and Eukaryotes: A Comparison
- Platypus DNA sequence is unique and essential for being a platypus.
- Prokaryotes can perform simultaneous transcription and translation due to lacking a nucleus and introns.
- Ribosomes attach to RNA as it is being transcribed in prokaryotes, allowing immediate protein synthesis.
- Eukaryotes separate transcription and translation due to having a nucleus, leading to a more complex process.
- Prokaryotes can produce multiple proteins from a single mRNA, enhancing gene expression efficiency.
- Prokaryotes utilize operons to code for multiple enzymes on a single mRNA, streamlining metabolic pathways.
- Eukaryotes have multiple RNA polymerases for different RNA types, increasing complexity compared to prokaryotes.
- Eukaryotic transcription initiation involves numerous transcription factors binding to DNA and RNA polymerase.
- Eukaryotic mRNA undergoes processing, including adding a 5' cap and a poly-A tail for stability and export.
- Eukaryotic pre-mRNA contains coding exons and non-coding introns, which are precisely removed during processing.
01:13:15
Importance of Precision Cutting in Genetic Processes
- Precision cutting is essential to ensure that base 104 aligns with the edge of the dark pink region and base 105 aligns with the end of the dark pink region.
- Each base added requires an ATP worth of energy, emphasizing the importance of efficient cutting.
- Intron-exon models in eukaryotic kingdoms suggest advantages, such as downstream regulatory elements involving introns.
- Introns may protect DNA against mutations caused by factors like UV light, enhancing DNA survival and consistency.
- Alternative splicing allows for the creation of multiple proteins from a single pre-mRNA by cutting exons and introns differently.
- Alternative splicing significantly increases protein diversity, enabling the production of various proteins from one gene.
- Prokaryotic cell translation involves the formation of an initiation complex with ribosomal subunits, tRNA, and mRNA.
- The ribosome advances by one codon, releasing tRNA carrying formal methionine at the E site and adding new amino acids to the growing polypeptide chain.
- Translation elongation continues until a stop codon is reached, terminating protein synthesis.
- TRNA, with its anticodon and amino acid attachment by aminoacyl tRNA synthetase, plays a crucial role in transferring amino acids during translation.
01:31:40
RNA Cloverleaf Structure and Protein Synthesis
- Cloverleaf structure in RNA is stable due to base pairing, creating a stable form.
- Focus on the acceptor end for binding amino acids and the anticodon for codon pairing.
- Example: Anticodon AUG pairs with codon UIC through base pairing.
- Aminoacyl tRNA synthetase ensures correct pairing of amino acids with tRNA.
- Enzyme reads anticodon to ensure accurate amino acid pairing, crucial to prevent mutations.
- TRNA with correct amino acid goes to ribosome for protein synthesis.
- Ribosome lacks ability to verify correct amino acid; responsibility lies with aminoacyl tRNA synthetase.
- Ribosome has two subunits, large and small, with distinct roles in protein synthesis.
- Initiation of translation in prokaryotes involves TRNA with methionine, small subunit, and mRNA.
- Elongation in translation involves adding new amino acids to the growing protein chain in the ribosome.
01:49:42
Protein Translation and Mutation: Key Concepts
- The release Factor protein hydrolyzes the protein at the stop codon, disassembling the ribosome.
- The large and small ribosomal subunits can jump to another mRNA to make more proteins.
- Messenger RNA lifespan determines how many times it can be translated.
- Sequences at the 5' and 3' ends of mRNA regulate protein production.
- Proteins destined for the rough ER are guided there by a signal recognition particle.
- Translation starts in the cytosol but can move to the rough ER for completion.
- Proteins are directed to specific cellular locations based on signal sequences.
- Mutation can lead to point mutations, affecting single bases in DNA.
- Point mutations can be silent, missense, or nonsense, altering protein structure.
- Frame shift mutations can create entirely new proteins by shifting the reading frame.
02:07:33
Genetic mutations impact evolution and ancestry.
- A frameshift mutation occurs when there is an addition or deletion of three bases, maintaining the reading frame.
- Chromosomal changes can occur through mechanisms like crossover, leading to deletions or duplications.
- Crossover between non-homologous chromosomes can result in genetic changes, impacting survival.
- Mutations are rare due to proofreading mechanisms, with relevance only if they affect the germ line.
- Evolutionary time allows unlikely mutations to become prevalent over millions of years.
- Mutation rates can be used to estimate common ancestry and divergence timelines, aiding in evolutionary studies.
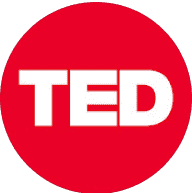
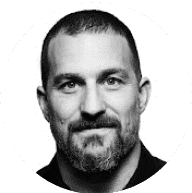
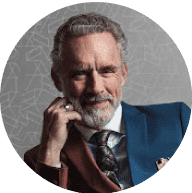

