Demystifying the Higgs Boson with Leonard Susskind
Stanford・2 minutes read
The text provides a detailed explanation of Higgs physics, emphasizing the role of quantum mechanics in understanding fields, particles, and mass generation in the Standard Model. It discusses concepts like field vibrations, condensates, Zilch charge, and the creation and decay of the Higgs boson through interactions with top quarks, highlighting the need for further research to explore potential new particles beyond the Standard Model.
Insights
- Fields, represented by vibrating particles, can have multiple values and energy levels, with the Mexican hat potential energy function illustrating spontaneous symmetry breaking and the uncertainty principle highlighting the probabilistic nature of charge in quantum physics.
- The Higgs boson, integral to the standard model, gives particles mass, with its decay probability favoring heavier particles like top quarks due to stronger coupling, and discrepancies in its decay into photons potentially indicating the presence of new particles challenging the existing model.
Get key ideas from YouTube videos. It’s free
Recent questions
What is Higgs physics?
The speaker explains the workings of Higgs physics.
Related videos
Wondrium
What Is the Higgs Boson? | Sean Carroll Discusses the God Particle
The Royal Institution
Sean Carroll - The Particle at the End of the Universe
PBS Space Time
The Equation That Explains (Nearly) Everything!
PBS Space Time
Could the Higgs Boson Lead Us to Dark Matter?
e-penser 2.0
Le bestiaire des particules - Quickie 28
Summary
00:00
Deciphering Higgs Physics: Fields, Vibrations, and Uncertainty
- The speaker aims to explain the nuts and bolts of Higgs physics, focusing on how things work rather than the historical excitement surrounding the Higgs boson's discovery.
- Quantum mechanics is essential to understanding Higgs physics, with the concept of quantization being crucial, particularly in angular momentum.
- Fields, such as electric or magnetic fields, are conditions in space that can affect the behavior of particles, with the vacuum typically having zero field energy.
- Fields can vibrate, with these vibrations representing particles or quanta of the field, and the energy of a field can be plotted as a function of its value.
- Fields can have multiple relevant values, and their energy can be represented in a two-dimensional plot, with the field's behavior resembling oscillations or particles.
- The concept of a Mexican hat potential energy function is introduced, where the field's lowest energy state is not at zero but at the brim of the hat, leading to a condensate or spontaneous symmetry breaking.
- The uncertainty principle dictates that empty space cannot have zero charge due to the uncertainty in the field's position and momentum, leading to a region of space with an uncertain amount of charge.
- An extra charged particle thrown into this space would displace the charge by one unit, illustrating the uncertainty and probabilistic nature of charge in quantum physics.
19:47
"Condensates, Superconductors, and Standard Model Particles"
- A condensate is a configuration of space where the amount of charge is uncertain, making it impossible to detect if an extra charge is added or removed.
- Superconductors exhibit behavior similar to condensates, where the charge is completely uncertain.
- The standard model in particle physics involves elementary particles with mass ranging from zero to a maximum known as the Planck mass.
- Ordinary particles like electrons, photons, and quarks have masses significantly lower than the Planck mass.
- The standard model includes fermions (matter particles like electrons and quarks) and bosons (force carrier particles like photons and gluons).
- The Higgs boson is believed to give particles mass, with all standard model particles having zero mass in theory.
- In the standard model, fermions can emit photons, gluons, and Z bosons, while bosons like photons and gluons play roles in interactions between particles.
- Fields can affect the mass of particles, as demonstrated by the example of water molecules in an electric field.
- Fields can create mass differences between particles by influencing their energy levels.
- Fields, like electric fields, can be represented as a condensate of photons, where the polarization of photons determines the direction of the field.
38:07
"Mass Acquisition in Particle Physics Explained"
- Water molecules emit and absorb photons, which are represented by green color; extra photons do not affect the molecule.
- The movement of a dumbbell through an electric field involves constant emission and absorption of photons, affecting the energy of the dumbbell's configurations.
- Various particles in nature can have mass without the need for the Higgs phenomenon, such as a box filled with massless radiation exhibiting mass due to energy.
- The mass of a proton, made of massless quarks and gluons, arises from the kinetic energy of these particles within the proton.
- The Dirac theory explains the mass of electrons, with mass being proportional to the rate at which electrons flip between left and right-handed spins.
- Electrons emit Z bosons when accelerating, which carry a separate charge called zilch; left and right-handed electrons have different zilch values.
- The standard model's mathematics dictates that left and right-handed electrons cannot switch due to conservation of zilch, leading to the introduction of the Ziggs boson.
- The Ziggs boson interacts with the condensate to allow left and right-handed electrons to switch by emitting and absorbing zilch-carrying particles.
- The Z boson can absorb zilch from the condensate, transforming into a Ziggs particle, and vice versa, allowing for mass acquisition.
- The Higgs boson is associated with the condensate's density oscillations, creating compressional waves within the condensate, known as a Higgs boson.
56:59
"Deciphering Higgs Boson Decays: Unveiling Mysteries"
- The Higgs boson, denoted as H, can create various particles like electrons, positrons, quarks, and other fermions when it decays.
- The probability of Higgs decay is linked to the mass of the particle it decays into, with heavier particles being favored due to stronger coupling.
- The Higgs boson can be created by colliding electrons and positrons, but the lightness of these particles made Higgs production challenging.
- The Higgs boson prefers to decay into top quarks, which are significantly heavier than electrons, making them the most likely decay product.
- Creating top quarks in nature is difficult as they decay rapidly, necessitating the collision of high-energy gluons to produce them.
- Gluons, which couple quarks, are too light to create top quarks directly, requiring high-energy gluons colliding to form top quarks.
- The process of creating Higgs bosons involves gluons from protons colliding to produce top quarks, which then combine to form the Higgs boson.
- The discovery of the Higgs boson at the LHC confirmed the Standard Model's correctness, but a slight discrepancy in Higgs decay into photons hints at potential new particles beyond the model.
- The discrepancy in Higgs decay into photons could indicate the existence of undiscovered particles, possibly supersymmetric, challenging the Standard Model.
- Monitoring the consistency of Higgs decays with the Standard Model, particularly into photons, is crucial for potentially uncovering new physics beyond the established model.
00:00
Explore Stanford University's website for details.
- Visit stanford.edu for more information.
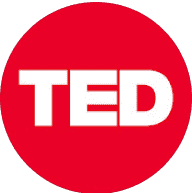
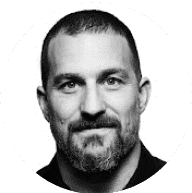
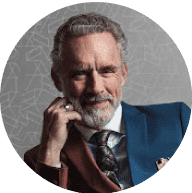

