d & f BLOCK in 1 Shot: All Concepts, Tricks & PYQs | NEET Crash Course | Ummeed
Competition Wallah・2 minutes read
The text discusses the complexities of transition metals, including properties, electron configurations, and oxidation states, emphasizing the importance of understanding these concepts for various elements like zinc, cadmium, and mercury. It delves into the significance of atomization enthalpy, melting points, and metal bonding in transition elements, highlighting key patterns and trends across the periodic table for better comprehension.
Insights
- The speaker emphasizes the importance of understanding the properties of D Block elements, particularly the transition metals and their characteristics.
- Specific examples like zinc, cadmium, and mercury are used to illustrate transition metals, highlighting the importance of oxidation states in determining their properties.
- The text delves into the relationship between ionization energy and achieving different oxidation states, emphasizing the complexities involved in elements like chromium, copper, and zinc.
- The stability of oxidation states in transition metals, such as the preference for +2 states in zinc and copper, is discussed, showcasing the significance of hydration energy in stabilizing these states.
- The text explores the catalytic properties of D-block elements, with iron and titanium commonly used in reactions, explaining how catalysts interact with reactants to lower activation energy.
- Lanthanide contraction is explained, detailing the decrease in size of elements in the 4D and 5D series, with a focus on europium's role in maintaining size and the unique electronic configurations of lanthanides and actinides.
Get key ideas from YouTube videos. It’s free
Recent questions
What are transition metals?
Transition metals are elements found in the d-block of the periodic table, characterized by their partially filled d orbitals. These metals exhibit a wide range of oxidation states and are known for their high tensile strength, thermal and electrical conductivity, ductility, and malleability. Examples include zinc, cadmium, and mercury, which illustrate the properties of transition metals.
How do transition metals bond?
Transition metals form weak metal-metal bonds due to the presence of unpaired electrons in their d orbitals, favoring interatomic interactions. This leads to strong metal bonding, resulting in properties like high melting and boiling points. For example, mercury remains liquid at room temperature due to its weak metal-metal bonding. The atomization enthalpy of transition metals correlates with their melting points, indicating the strength of metal bonding.
Why do transition metals have variable oxidation states?
Transition metals exhibit variable oxidation states due to the presence of unpaired electrons in their d orbitals, allowing for different electron configurations. The transition from one oxidation state to another involves electron transfer, with stability achieved through synergic bonding. Understanding the reasons behind variable oxidation states is crucial for exams like NEET, as different states impact the reactivity and properties of transition metals.
What is the significance of ionization energy in transition metals?
Ionization energy plays a crucial role in determining the oxidation states of transition metals. Elements like chromium, copper, and zinc face challenges in achieving certain oxidation states due to their electron configurations. The stability of oxidation states is influenced by factors like hydration enthalpy, with transitions between states indicating changes in reactivity and properties. Ionization energy impacts the ease of achieving different oxidation states, highlighting the complexities involved in the process.
How do transition metals act as catalysts?
Transition metals exhibit catalytic properties, with elements like iron and titanium commonly used in various reactions. Catalysts interact with reactants by either reacting on the surface or being adsorbed on the reactant's surface, reducing activation energy. The presence of a catalyst enhances bond formation between reactant molecules, utilizing d electrons to increase reactant concentration on its surface. Understanding the role of transition metals as catalysts is essential in facilitating chemical reactions and increasing reaction rates.
Related videos
YAKEEN
D & F BLOCK ELEMENTS in 1 Shot: FULL CHAPTER COVERAGE (Concepts+PYQs) || Prachand NEET
Lakshya JEE
D & F Block FULL CHAPTER | Class 12th Inorganic Chemistry | Lakshya JEE
JEE Wallah
PERIODIC TABLE in 60 Minutes || Full Chapter Revision || Class 11th JEE
UDAAN
Metals And Non Metals | Complete NCERT WITH BACK EXERCISE in 1 Video | Class 10th Board
The Organic Chemistry Tutor
How To Calculate Oxidation Numbers - Basic Introduction
Summary
00:00
"Exploring D Block Elements and Transition Metals"
- The text involves a conversation with children about upcoming events like Holi and studying.
- The speaker encourages interaction and engagement with the audience, urging likes and subscriptions.
- The discussion transitions to a lesson on D Block elements in chemistry.
- The speaker emphasizes the importance of understanding the properties of D Block elements.
- Details about the electron configuration and series of D Block elements are explained.
- The text delves into the transition metals and their characteristics.
- Specific examples like zinc, cadmium, and mercury are used to illustrate transition metals.
- The importance of oxidation states in determining transition metals is highlighted.
- The text concludes with a detailed explanation of how certain elements qualify as transition metals based on their electron configurations.
- The speaker uses a family analogy to simplify the concept of transition metals and their oxidation states.
15:23
Transition Elements: Confined, Named, and Stable
- Silver's limit is 410, transitioning to Silver D10 in the plus two state.
- Transition elements are fully fed, including grandfather, father, and grandson.
- The compound formation in Silver Plus Two is confirmed.
- The naming of transition elements is attributed to the work of the grandson.
- The confining of elements in the periodic table is discussed, focusing on D Block elements.
- General electronic confining involves filling n-1 d with 1 to 10 electrons and ns2.
- Exceptions in confining, like ns1 and ns0, can lead to confusion.
- Specific elements like chromium, copper, and others exhibit unique confining properties.
- Physical properties of transition elements include high tensile strength, thermal and electrical conductivity, ductility, and malleability.
- Zinc, cadmium, and mercury are stable transition elements with unique properties.
30:29
Transition Metal Melting Points and Bonding
- Weak metal-metal bonding leads to low melting and boiling points.
- Mercury remains liquid at room temperature due to its weak metal-metal bonding.
- Manganese, with its half-filled d5 stable configuration, has low tendency to participate in bonding.
- Tungsten has the maximum melting point in the 5d series of transition elements.
- Maximum melting point in the 3D series is chromium, while zinc has the minimum melting point.
- Unpaired electrons in d orbitals favor interatomic interactions, leading to strong metal-metal bonding.
- Atomization enthalpy correlates with melting point, indicating strong metal bonding.
- The melting point pattern in transition elements follows a specific order from the 3rd to the 12th group.
- Copper, gold, and silver in the 11th group have low melting points, making copper ideal for electric wires.
- Manganese and technician have hexagonal close packing (HCP) and body-centered cubic (BCC) structures in the solid state.
45:30
Metals, Enthalpy, and Periodic Table Essentials
- In the 3D series, Chromium drops after Chromium Manganese drops and grows again.
- Atomization enthalpy is crucial in reactions, converting solid metal to gas through sublimation.
- Ionization enthalpy is essential in the journey of metal ions, with two ionization energies for Metal Plus 2.
- Metals with high atomization enthalpy tend to remain noble, as per NCERT's explanation.
- The size comparison within the group reveals a decrease from left to right and an increase from top to bottom.
- The 5D series and 4D series almost equalize due to Lanthanide contraction, affecting their sizes.
- Lanthanide contraction causes elements to become smaller, leading to almost equal sizes in the 5D and 4D series.
- Understanding the periodic table and the impact of D&F blocks is crucial for answering related questions.
- Continuous learning from one teacher throughout is advised for a better understanding of complex topics.
- The periodic table's detailed explanations and the importance of specific series are reiterated throughout the chapter.
59:43
"Element Size, Properties, and Ionization Energy"
- Size of elements ordered according to Zarco rules
- Hafnium's properties questioned for similarity
- Explanation of similar properties in elements
- Discussion on elements belonging to D block
- Explanation of lanthanide contraction and shielding of electrons
- Relationship between Z effective and shielding
- Explanation of lanthanoid contraction and its effects
- Differentiation between ordinary and extraordinary transition series
- Increase in density from titanium to copper
- Order of ionization energy in transition series
01:14:49
Understanding Ionization Energy and Oxidation States
- The text discusses the content of NCERT books for children, focusing on the concept of ionization energy and the difficulty in achieving certain oxidation states.
- It mentions the importance of ionization energy in the process of achieving different oxidation states, particularly in elements like chromium, copper, and zinc.
- The text explains the relationship between ionization energy and the ease of achieving different oxidation states, emphasizing the challenges faced in reaching certain states.
- It delves into the specifics of ionization energy in elements like chromium and copper, highlighting the complexities involved in the process.
- The text discusses the rarity of certain oxidation states, such as manganese in +3, and the stability of oxidation states like copper in +2.
- It explains the significance of energy gaps between electron orbitals in determining oxidation states and the role of hybridization in facilitating electron movement.
- The text touches on the variety of oxidation states exhibited by elements like manganese, scandium, and zinc, emphasizing the stability of certain states over others.
- It mentions the lack of variable oxidation states in elements like scandium and zinc, contrasting them with elements that exhibit a wider range of oxidation states.
- The text concludes by highlighting the trend of increasing and decreasing variety in oxidation states across the periodic table, emphasizing the stability of certain states over others.
- It underscores the importance of understanding the patterns and trends in oxidation states to grasp the behavior of elements across the periodic table.
01:28:54
Variable oxidation states in elements explained concisely.
- Variable oxidation states tend to be higher at the beginning and end of a series.
- The availability of oxidation states is lower at extreme ends due to fewer electrons at the beginning.
- The number of electrons impacts the variety of oxidation states, with fewer electrons at the start.
- The ending of a series has more electrons but fewer orbitals for bonding.
- Scandium does not show a +2 oxidation state, while Titanium's +3 and +2 are not stable.
- Zinc is stable at +2, similar to Copper's stability at +1.
- The stability of oxidation states increases from top to bottom in a series.
- Chromium +6 acts as an oxidant due to its less stable nature.
- Low oxidation states of metals can be stabilized through synergic bonding.
- Understanding the reasons behind variable oxidation states is crucial for exams like NEET.
01:42:30
Transition metals, alloys, and catalytic properties explained.
- Moline and tungsten have a +6 oxidation state, not acting as oxidizing agents.
- Lower oxidation states of metals can be stabilized through synergic bonding.
- Maximum oxidation state is determined by the sum of s electrons and (n-1) d electrons.
- Manganese's maximum oxidation state is reached when all five d electrons are unpaired.
- Zinc is not a transition element, unlike manganese.
- Alloys are formed by mixing metals with similar sizes to enhance strength and practical use.
- Important alloys include brass (copper and zinc), German silver (copper, zinc, and nickel), and bronze (copper and tin).
- Alloys are hard, have high melting points, and are designed for strength without excessive weight.
- Interstitial compounds involve trapping small elements in the gaps of metal lattices, increasing hardness and conductivity.
- D-block elements exhibit catalytic properties, with iron commonly used in ammonia production and titanium in polymerization.
01:59:04
Catalyst enhances reactions by weakening bonds.
- Catalyst interacts with reactants by either reacting on the surface or being adsorbed on the reactant's surface.
- The catalyst and reactant interactions involve high activation energies, necessitating the introduction of an agent from the D block to divert attention.
- The agent's role is to distract the reactants from their original bonds, weakening them and reducing activation energy.
- The catalyst's presence on a solid surface enhances bond formation between reactant molecules.
- The catalyst utilizes 3d and 4d electrons to increase reactant concentration on its surface.
- The catalyst's effect is to strengthen bonds between reactants and atoms on its surface, particularly with metals.
- The catalyst's role is crucial in evolving bond formation between reactant molecules.
- The process involves a mediator with a variable oxidation state, aiding in the reduction of activation energy.
- Iron plays a significant role in reduction reactions, transitioning between iron +3 and +2 oxidation states.
- Standard reduction potential guides the reactivity of elements in the 3D series, with copper and zinc showcasing distinct behaviors in liberating H2 gas from acids.
02:16:02
Chemical reactions and electron transfer processes explained.
- Transition from zero to two in the series involves electron transfer and the formation of H2 gas with acid.
- Copper atomization leads to Copper plus two, with the electron transfer process explained.
- A&B not balanced by C indicates the need for balance in reactions.
- Concentrated H2SO4 and HNO3 should not be mixed due to their oxidizing properties.
- Zinc's electron transfer process to form H2 gas is detailed.
- Copper does not produce H2 gas, emphasizing the need to handle it carefully.
- Copper can oxidize with strong oxidants like concentrated H2SO4 and HNO3.
- The stability of half-filled orbitals in transition metals is discussed.
- The impact of ionization energy on Copper's properties is explained.
- The significance of hydration energy in stabilizing Copper at plus two is highlighted.
02:31:42
Transitioning Metal Oxidation States for Stability
- Stability at +2 is preferred over coming to zero due to high hydration enthalpy.
- High hydration enthalpy leads to stability in the plot, causing a transition from +2 to zero.
- Negative value indicates higher stability at +2, avoiding transition to zero.
- Scandium +3 prefers to stay in +2 due to noble gas confinement at zero.
- Zinc transitions from +3 to +2 at midnight to achieve d10 configuration.
- Manganese +3 acts as an oxidant, transitioning to +2 for stability.
- Iron +3 to +2 transition involves a less positive value, indicating a gradual change.
- Comparison of iron and manganese oxidation states reveals stability at +2 for d5 configuration.
- Nedian plot shows a less negative value for the transition from +3 to +2.
- pH-based conversion between dichromate and chromate ions is not a redox change, influenced by medium acidity or basicity.
03:04:36
Chromium Ion Structure and Bonding Characteristics
- Chromate ion structure: 2 minuses, 2 singles, 2 doubles, with chemical bonding.
- Tetrahedral structure: Chromium with 4 oxygen atoms, forming sp3 hybrid bonds.
- Formal charge calculation: Average formal charge of -2/4, with 6/4 bond orders.
- Partial double bonds: Equal number of bonds with partial double bond character.
- Color of chromium: Diamagnetic if chromium is 0, colorless for d0 and d10 configurations.
- Ligand to Metal Charge Transfer (LMCT): Explanation of LMCT and its impact on color.
- Chromate dye structure: Double bonds, resonance, and partial double bond character.
- Bond lengths: Terminal CO bonds have different lengths due to resonance.
- Resonance stability: Non-equivalent resonance and bond angle explanation.
- Primary vs. secondary standards: Na2Cr2O7 as a secondary standard due to solubility and color properties.
03:20:18
"NCRT: Magnetism, Bonding, and Oxidation Reactions"
- NCRT mentions temperature-based weak paramagnetism and diamagnetism in the syllabus
- Magnetism and temperature-dependent paramagnetism are discussed
- The structure of mno4 2- and its bond order are detailed
- The bond lengths and differences between magnet ion and paramagnet ion are explained
- Preparation of kmno4 involves oxidizing mno2 with k3 or in acidic medium
- The laboratory method for preparing kmno4 is outlined
- Thermal decomposition of kmno4 results in potassium changing to magnet and mno2
- Oxidizing properties of mno4- and dichromate ions are highlighted
- The relation between chromate and cl2 as oxidants is discussed
- Oxidation reactions in acidic and weak basic mediums are compared, emphasizing iodate formation in basic medium
03:38:11
Chemical reactions and color changes in solutions.
- P2 gas does not react with magnets or dyes, but the effect of magnet on SO2 gas can decolorize and change chromate to orange.
- Chromium in +3 state creates a green compound in a basic medium, turning orange to yellow with increased pH.
- In a basic medium, chromate converts to chromate, changing color to orange or yellow.
- To maintain a basic medium, remove O- ions to prevent the reaction from reverting to acidic.
- Acidify the medium using HClO2 to prevent the reaction from going back to basic.
- MNO4- can change oxidation states, indicating changes in reactions.
- Ferrous ammonium sulphate can transition from +2 to +3 oxidation states.
- Mohr Salt preparation involves creating an acidic medium using dilute H2SO4.
- Concentrated H2SO4 acts as a dehydrating agent, removing water from compounds.
- Dilute H2SO4 is used to prevent explosive reactions in compounds like Mn2O7.
03:53:55
Chemical reactions and properties of elements.
- KMnO4 and water reaction is positive, with KMnO4 acting as an oxidizer.
- Analyzing the reaction analytically involves using reagents and Accusys.
- The reaction is kinetically slow but can be accelerated by avoiding contact between the substances.
- Thermodynamics supports KMnO4 oxidation, but the rate of reaction is slow.
- Ethyl alcohol reacts with potassium dichromate, changing color from orange to green.
- Fluorine and oxygen's higher oxidation states are stabilized due to electronegativity.
- Oxygen's ability to form multiple bonds with metals stabilizes higher oxidation states.
- V2O5 exhibits amphoteric behavior, reacting with both acids and bases.
- Lanthanides and actinides have unique electronic configurations, with exceptions like thorium.
- Remember the periodic table mnemonic "Cinema Gadar Looted" for lanthanides and actinides.
04:14:21
Lanthanides: Size, Electron Configuration, and Properties
- Lanthanide contraction leads to a decrease in size, with europium being a key element to consider for maintaining size.
- The imperfect building of 4f electrons in lanthanides causes a continuous decrease in size, especially in the plus three state.
- Ionization energy in lanthanides shows a pattern where the third electron is released quickly to achieve inert gas configuration.
- Common oxidation states in lanthanides, particularly plus three, exhibit stability and specific characteristics, such as cerium acting as a strong oxidant.
- Lanthanides in the plus three state are generally colored, with cerium being a notable exception as it is colorless, and most lanthanides exhibit magnetic properties, except for cerium and ytterbium in certain configurations.
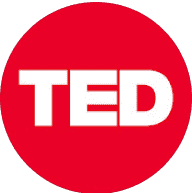
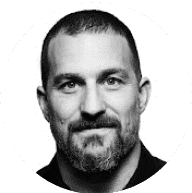
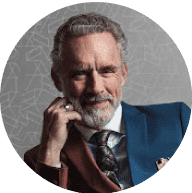

