Coordination Compounds Detailed Oneshot + PYQ Chapter 5 Class 12 Chemistry CBSE 2024-25 #cbse.
Sourabh Raina・2 minutes read
Coordination compounds involve transition metals forming complexes with ligands like chlorophyll, hemoglobin, and Vitamin B12, with various practical applications such as electroplating and medicinal chemistry. Werner's theory explains the primary and secondary valence in coordination compounds, while different ligands and isomerism types influence the structure and characteristics of these complexes.
Insights
- Coordination compounds involve transition metals forming complexes with ligands, essential for compounds like chlorophyll, hemoglobin, and Vitamin B12.
- Practical applications of coordination compounds range from electroplating to medicinal chemistry, showcasing their diverse utility.
- Werner's theory explains primary and secondary valences in coordination compounds, crucial for understanding bonding mechanisms.
- Isomerism in coordination compounds, including stereo and structural isomerism, leads to diverse spatial arrangements and properties.
Get key ideas from YouTube videos. It’s free
Recent questions
What are coordination compounds?
Coordination compounds involve transition metals forming complexes with ligands.
Related videos
Munil Sir
coordination compounds one shot revision class 12th chemistry, coordination compounds one shot
NCERT Wallah
COORDINATION COMPOUNDS | Complete Chapter in 1 Shot | Class 12th Board - NCERT
Cognito
GCSE Chemistry - What is an Ionic Compound? Ionic Compounds Explained #15
Tyler DeWitt
Ionic vs. Molecular
Competition Wallah
d & f BLOCK in 1 Shot: All Concepts, Tricks & PYQs | NEET Crash Course | Ummeed
Summary
00:00
"Transition metals form stable coordination compounds"
- Coordination compounds involve transition metals from the D block forming complexes with atoms and ions bound to a specific number of ligands.
- Biologically important compounds like chlorophyll, hemoglobin, and Vitamin B12 are coordination compounds containing magnesium, iron, and cobalt, respectively.
- Practical applications of coordination compounds include electroplating, dyeing, medicinal chemistry, metallurgical processes, catalysts, and analytical reagents.
- Werner's theory of coordination compounds explains primary valence as ionizable and secondary valence as non-ionizable, satisfied by neutral and negative ions.
- Coordination numbers determine the arrangement of ligands around a central metal atom, with different coordination numbers leading to various structures like octahedral and tetrahedral.
- Coordination entities consist of a central metal atom/ion bound to a fixed number of ions and molecules in a specific geometric arrangement.
- Ligands are molecules or ions that donate electron pairs to form coordinate bonds with central metal atoms/ions, with different types like monodentate, bidentate, and polydentate based on the number of donor sites.
- Polydentate ligands like ethylenediamine tetraacetate can form multiple coordinate bonds with central metal atoms/ions through multiple donor sites.
- Some ligands, like nitrite, can be unidentate, donating electron pairs through a single donor atom, either nitrogen or oxygen.
- The identity of coordination compounds remains stable in solid form and aqueous solutions, with counter ions dissociating in double salts but not in coordination compounds.
17:43
"Chelate Ligands and Coordination Numbers Explained"
- Ligands can form bonds with the left side, and if they bond with the metal on the left side, it is called a dented ligand.
- Only one person can make this bond, and it is a uni-dentated bond.
- A negative charge from the sulfur side can donate an electron pair to the metal, while from the nitrogen side, it is called isothio cyanate.
- Coordination through sulfur and nitrogen atoms is termed as dented ligand, and it is uni-dentated.
- Chelate ligands are more stable and form a ring-like structure known as a chelate ring, with an example being PCl2.
- A di-amide has a ring-like structure, forming a chelate ring, which enhances stability.
- Chelation increases stability, and the more chelate rings present, the more stable the structure becomes.
- The coordination number indicates the total number of ligands donor atoms directly attached to the metal ion.
- The coordination sphere includes the central atom, ion, and ligands enclosed in square brackets, representing the coordination entity.
- The oxidation number of the central atom is defined as the charge it carries when all ligands and shared electron pairs are removed.
33:58
Coordination Compounds: Naming, Formulas, Isomerism
- Indicate the number of individuals in the coordination entity if more than one
- Ligand presence leads to death, but Tetra Extra is used by these individuals
- Die Tri Tetra is the order of ligands alphabetically
- Amica Aqua precedes Chlorido in alphabetical order
- Tetra Amica A is written if Amaze is present, and Tetra Amica Chlorido Cobalt Aussie is the result
- Calculate the oxidation number by considering cl2 and cl3
- Water is neutral, with an overall charge inside cl-1 of +2 x +3
- Use numerical prefixes like Die, Bis, Tris, Tetra Kiss when multiple ligands are present
- Determine the oxidation number of Cobalt in Cobaltate
- Name the coordination entity based on its charge and complexity
- Identify the name of the ligand in MB Dented Leagues
- Formulas of coordination entities are structured with the central atom first, followed by ligands alphabetically
- Include ligand abbreviations in brackets for polyatomic ligands
- Charge of coordination entities is indicated outside square brackets
- Balance the charge of cations and anions in coordination entities
- Write the formula for Potassium Tetra Hydroxy Zincate
- Determine the formula for Hexa Amaze Platinum Fur Chloride
- Find the charge in the brackets for Chromium and Iron coordination compounds
- Discuss isomerism in coordination compounds, including stereo and structural isomerism
- Stereoisomers have the same chemical formula but different spatial arrangements of atoms
- Stereoisomers can be classified into geometrical and optical isomerism
48:47
Isomerism in Complex Coordination Compounds
- Different types of isomerism arise in complex compounds due to the presence of different ligands.
- Isomerism occurs when the geometric arrangement of ligands changes with respect to each other.
- Complex isomerism is observed when the coordination number is four.
- Complexes with four donor atoms associated with the metal exhibit Heddle and Square Planar Geometry or Tetra Heddle Geometry.
- Tetra Heddle complexes do not show geometric isomerism if the relative position of atoms remains the same.
- Planar mx2 l2 x complexes can form cis or trans isomers based on the arrangement of ligands.
- A A A ligands can form two cis and one trans isomer based on the position occupied by the ligand.
- Complexes with a coordination number of six can exhibit cis and trans isomers based on the arrangement of ligands.
- Octahedral Complex Leagues evolve optical isomers, with A aa3 representing a dye-bound ligand.
- Structural isomers in coordination compounds include linkage isomers, coordination isomers, ionization isomers, and solvent isomers.
01:05:05
Coordination Compounds: Isomers, Bonds, Geometries, Properties
- Structural isomers are discussed, with adjacent and opposite forms leading to transformations.
- Valence bond theory is introduced as the primary focus in coordination compounds.
- Metal atoms and ions are identified as the entities forming bonds under the influence of ligands.
- Hybridized orbitals, including 3d, 4s, 4p, and 4d, are utilized in bonding.
- The number of bonds ligands make is determined by the hybridized orbitals.
- Specific geometries like tetrahedral and square planar are formed based on the hybridized orbitals.
- Strong and weak ligands are differentiated based on their electron pairing and repulsion effects.
- Examples from NCRT are used to illustrate the concepts discussed.
- Magnetic properties of coordination compounds are explained, with diamagnetic and paramagnetic behaviors detailed.
- Crystal Field Theory is contrasted with Valence Bond Theory, emphasizing the electrostatic attraction between metal ions and ligands.
01:21:44
Crystal Field Theory in Transition Metal Complexes
- Metal atoms or ions are surrounded by spheres, with negative charges leading to equal repulsion in d orbitals.
- Repulsion between metal d orbitals and ligands causes degeneration, affecting energy levels.
- Crystal field splitting occurs in octahedral coordination entities due to repulsion between ligands and metal d orbitals.
- Strong field ligands cause larger splitting, while weak field ligands result in lesser splitting.
- The Spectro Chemical series arranges ligands based on increasing field strength.
- Electron distribution in d orbitals follows specific patterns based on crystal field splitting and pairing energy.
- Crystal field splitting energy, represented by delta, determines the energy levels of d orbitals in tetrahedral complexes.
- Tetrahedral complexes exhibit less energy release, leading to unpaired electrons in high spin configurations.
- The energy difference in tetrahedral complexes, represented by delta t, is 4/9 of delta in octahedral complexes.
- In tetrahedral complexes, the energy release is insufficient for pairing, resulting in high spin configurations with unpaired electrons.
01:35:50
Color and Bonding in Coordination Compounds
- In crystal field theory, if delta n is less than p, electrons go to t2g due to lower energy than pairing energy, making pairing difficult.
- For d4 ions, if delta n is greater than p, electrons may go to t2g orbital, allowing pairing due to higher energy.
- Low spin tetrahedral complexes do not pair electrons due to insufficient orbital splitting energy, resulting in no pairing.
- Transition metal coordination compounds exhibit a wide range of colors due to absorption of specific wavelengths from white light, reflecting the remaining colors.
- The color of coordination compounds is explained by crystal field theory, attributing it to DD transitions where electrons move between d orbitals.
- Octahedral complexes follow crystal field theory, with t2g and eg levels splitting, allowing electrons to transition between these levels upon absorbing light.
- Different ligands near the central metal ion in complexes create varied crystal field splittings, leading to different colors based on absorbed radiation wavelengths.
- Changing ligands in water-to-chloride ratios in nickel complexes alters colors, with different ligands producing distinct crystal field splittings and resulting colors.
- Metal carbonyls form unique structures based on ligands like carbonyls, with varying metal-carbon sigma and pi bonds influencing colors and properties.
- Coordination compounds are analyzed qualitatively and quantitatively through color reactions, classical and instrumental methods, and selective estimation of metal ions.
01:51:48
Metal Coordination Compounds: Formation, Decomposition, and Uses
- Coordination compounds are formed from metals and can be decomposed to obtain pure metal. By converting the compound to NiCO4, pure nickel can be obtained. Washing the developed film with hypo solution dissolves the un decomposed AgBr to form a complex. Chelate ligands like de penis amine and des ferroc sine b can be used to remove toxic proportions of metals like copper and iron. Platinum complexes are used in medicinal chemistry for treating lead poisoning.
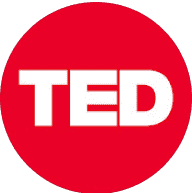
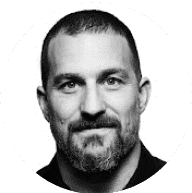
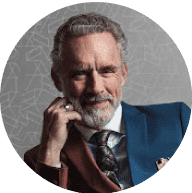

