Chemical Bonding: COMPLETE Chapter in 1 Video | Quick Revision | Class 11 Arjuna JEE
Arjuna JEE・2 minutes read
The lecture emphasizes the importance of understanding chemical bonding and molecular structures for successful study in chemistry, detailing concepts such as ionic and covalent bonds, lattice energy, and hybridization. It also highlights how factors like electronegativity, bond angles, and the presence of hydrogen bonding significantly influence the physical properties and behavior of molecules.
Insights
- The lecture emphasizes the critical importance of understanding "Chemical Bonding and Molecular Structures" for students, as a solid grasp of this topic is foundational for success in both inorganic and organic chemistry.
- Students are encouraged to actively engage with the material, especially those who have not yet read the chapter or require a revision, to prevent future confusion in their chemistry studies.
- Chemical bonds are described as the attractive forces that hold atoms or molecules together, primarily formed to achieve stability by lowering energy levels, often targeting a noble gas electron configuration with eight electrons in the outer shell.
- Different types of chemical bonds are introduced, including ionic bonds, which involve electron transfer, and covalent bonds, characterized by electron sharing. The lecture also highlights the significance of lattice energy in ionic bonds, noting that stronger ionic interactions arise from higher charges and shorter distances between ions.
- Lewis Theory is presented as a method for visualizing bonding through Lewis Dot Structures, which help illustrate the arrangement of valence electrons and the octet rule, while also addressing exceptions like hypovalent and hypervalent species.
- The lecture discusses molecular orbital theory, which provides a more comprehensive understanding of bonding by examining the combination of atomic orbitals to form molecular orbitals, emphasizing how bond order and the presence of unpaired electrons influence molecular stability and properties.
- Hydrogen bonding is explored as a significant intermolecular force that affects the physical properties of substances, with its strength being influenced by the electronegativity of involved atoms, and highlights how this concept relates to solubility and boiling points in various compounds.
Get key ideas from YouTube videos. It’s free
Recent questions
What is a chemical bond?
A chemical bond is the force that holds atoms or molecules together, allowing them to form stable compounds. This stability is achieved through various types of bonds, such as ionic, covalent, and coordinate bonds, each characterized by different mechanisms of electron interaction. For instance, ionic bonds occur when electrons are transferred from one atom to another, typically between metals and nonmetals, resulting in the formation of charged ions. Covalent bonds, on the other hand, involve the sharing of electrons between atoms, allowing them to achieve a full outer shell of electrons, which is crucial for stability. Understanding chemical bonds is fundamental in chemistry, as they dictate the properties and behaviors of substances.
How do ionic bonds form?
Ionic bonds form through the transfer of electrons from one atom to another, resulting in the creation of charged ions. Typically, this process occurs between metals, which tend to lose electrons and become positively charged cations, and nonmetals, which gain electrons to become negatively charged anions. The electrostatic attraction between these oppositely charged ions leads to the formation of a stable ionic compound. The strength of ionic bonds is influenced by factors such as the charges of the ions and the distance between them, with higher charges and shorter distances resulting in stronger bonds. This type of bonding is essential for the formation of many salts and minerals.
What is hybridization in chemistry?
Hybridization in chemistry refers to the process of mixing atomic orbitals to create new hybrid orbitals that are used in bond formation. The number of hybrid orbitals formed is equal to the number of atomic orbitals that mix. Different types of hybridization correspond to specific molecular geometries; for example, sp hybridization results in a linear shape, sp² leads to a trigonal planar arrangement, and sp³ gives a tetrahedral geometry. Hybridization is crucial for understanding the bonding and shape of molecules, as it helps explain how atoms can form multiple bonds and the angles between those bonds. This concept is fundamental in predicting molecular behavior and reactivity.
What is the significance of bond angles?
Bond angles are significant because they determine the spatial arrangement of atoms in a molecule, which in turn influences the molecule's shape and properties. The bond angle is primarily influenced by the hybridization of the central atom and the presence of lone pairs of electrons. For instance, in sp³ hybridized molecules, the bond angle is approximately 109.5°, while in sp² hybridized molecules, it is about 120°. The arrangement of electron pairs around the central atom minimizes repulsion, as described by the Valence Shell Electron Pair Repulsion (VSEPR) theory. Understanding bond angles is essential for predicting molecular geometry, which affects reactivity, polarity, and interactions with other molecules.
What are hydrogen bonds?
Hydrogen bonds are a specific type of intermolecular attraction that occurs when hydrogen is covalently bonded to highly electronegative elements such as fluorine, oxygen, or nitrogen. This bond creates a partial positive charge on the hydrogen atom and a partial negative charge on the electronegative atom, leading to an attraction between molecules. Hydrogen bonds are generally stronger than other types of van der Waals forces but weaker than covalent and ionic bonds. They play a crucial role in determining the physical properties of substances, such as boiling points and solubility. For example, water's high boiling point is largely due to the extensive hydrogen bonding between its molecules, which requires significant energy to break.
Related videos
Arjuna NEET
Chemical Bonding FULL CHAPTER | Class 11th Inorganic Chemistry | Arjuna NEET
Robin Barkes
2.1 FAU High Bio 2
ALLEN NEET
NEET 2024 🎯 Score 180/180 | Chemistry Top 20 Questions ➡️ Chemical Bonding and Molecular Structure
LearnoHub - Class 11, 12
Chemical Bonding Class11 One Shot | CBSE NEET JEE
CrashCourse
Carbon... SO SIMPLE: Crash Course Biology #1
Summary
00:00
Understanding Chemical Bonding and Stability
- The lecture focuses on the chapter "Chemical Bonding and Molecular Structures," which is essential for understanding both inorganic and organic chemistry, emphasizing that without this knowledge, students will struggle with further chemistry concepts.
- The session is designed for students who have either not read the chapter or need a thorough revision, encouraging them to engage with the material to avoid confusion later on.
- A chemical bond is defined as the force of attraction that holds particles, such as atoms or molecules, together, with stability being a key reason for bond formation.
- The lecture explains that atoms bond to achieve stability by reducing their energy levels, often aiming to attain a noble gas electron configuration, which is characterized by having eight electrons in the outermost shell.
- The types of chemical bonds discussed include ionic bonds, covalent bonds, and coordinate bonds, with ionic bonds formed through the transfer of electrons between metals and nonmetals, while covalent bonds involve the sharing of electrons.
- The concept of lattice energy is introduced, defined as the energy required to break one mole of an ionic lattice into gaseous ions, highlighting that higher charges and shorter distances between ions increase lattice energy.
- The lecture outlines the energy changes during ionic bond formation, noting that energy is absorbed when electrons are lost to form cations and released when the lattice structure is formed, indicating that stable bonds release more energy than they require.
- Lewis Theory is presented as a framework for understanding bond formation, where elements strive to achieve a full outer shell of electrons, either by gaining, losing, or sharing electrons.
- The formation of covalent bonds is explained through the sharing of electrons, with examples such as the Cl2 molecule, where two chlorine atoms share one electron each to achieve stability.
- The importance of understanding the factors affecting lattice energy is emphasized, particularly the dominance of charge over distance in determining the strength of ionic bonds, guiding students on how to approach related problems in chemistry.
12:29
Understanding Electron Pairs and Molecular Bonds
- The concept of lone pairs of electrons is introduced, emphasizing that both atoms in a covalent bond share an equal number of electrons, although the sharing may not always be equal in practice.
- The Lewis Dot Structure is crucial for visualizing the octet of each element, ensuring that the valence electrons are accurately represented; this is essential for understanding chemical bonding.
- A coordinate bond, also known as a dative bond, occurs when one atom donates both electrons to the bond, which can be represented with an arrow indicating the donor atom.
- The molecular structure of ozone is discussed, highlighting that it contains coordinate bonds and the importance of understanding formal charges to determine the stability of the structure.
- The formal charge of an atom can be calculated using the formula: Formal Charge = Valence Electrons - Non-bonding Electrons - (1/2) * Bonding Electrons, which helps identify the most stable Lewis structure.
- Limitations of the octet rule are addressed, noting that some elements, like boron, can be stable with fewer than eight electrons, leading to the concept of hypovalent species.
- Hypervalent species are introduced, where central atoms can have more than eight electrons in their valence shell, challenging the traditional octet rule.
- The Valence Bond Theory (VBT) is introduced as a new approach to explain molecular bonding, focusing on the overlap of atomic orbitals and the energy changes associated with bond formation.
- Types of orbital overlaps are categorized into positive, negative, and zero overlaps, with positive overlaps leading to sigma bonds and negative overlaps resulting in pi bonds.
- The strength of sigma bonds is greater than that of pi bonds due to the extent of orbital overlap, and the number of unpaired electrons in the participating orbitals determines the number of bonds an atom can form.
25:25
Understanding Hybridization and Molecular Geometry
- Hybridization refers to the intermixing of atomic orbitals to form new hybrid orbitals that are used in bond formation, with the number of hybrid orbitals formed equal to the number of atomic orbitals that mix.
- The types of hybridization include sp, sp², sp³, sp³d, sp³d², and sp³d³, with each type corresponding to specific geometries: linear for sp, trigonal planar for sp², tetrahedral for sp³, trigonal bipyramidal for sp³d, octahedral for sp³d², and pentagonal bipyramidal for sp³d³.
- To determine the hybridization of a molecule, count the number of electron pairs around the central atom, including both bond pairs and lone pairs, which will indicate the type of hybridization and geometry.
- For example, in NH₃ (ammonia), nitrogen has five valence electrons, three of which form bonds, resulting in one lone pair and four electron pairs, indicating sp³ hybridization.
- In PCl₅ (phosphorus pentachloride), phosphorus has five valence electrons, all of which are used in bond formation, leading to sp³d hybridization with five electron pairs and a trigonal bipyramidal geometry.
- The Valence Shell Electron Pair Repulsion (VSEPR) theory states that electron pairs around a central atom will arrange themselves to minimize repulsion, influencing the molecular geometry based on the number of lone pairs and bond pairs.
- The repulsion order is: lone pair-lone pair > lone pair-bond pair > bond pair-bond pair, affecting bond angles and molecular shape; for instance, a molecule with two bond pairs and one lone pair will have a bent shape.
- In cases where there are five electron pairs, such as in SF₄ (sulfur tetrafluoride), the geometry remains trigonal bipyramidal, but the shape becomes seesaw due to the presence of one lone pair.
- The bond order, which indicates the number of bonds between two atoms, can be calculated; for example, a single bond has a bond order of one, while a double bond has a bond order of two.
- Limitations of VSEPR theory include its inability to explain bond formation and differences in bond energy, which require further analysis through molecular orbital theory for a complete understanding of molecular behavior.
39:29
Understanding Chemical Bonds and Their Properties
- A double bond between oxygen is referred to as a "ratu" bond, while nitrogen has a triple bond, making it stronger than a double bond, which is stronger than a single bond.
- The bond order for isoelectronic species is equal, and for O2, there are 18 electrons, resulting in a bond order of 2, while F2 has a single bond and also has 18 electrons, giving it a bond order of 1.
- Resonance structures are used to explain molecules that cannot be represented by a single structure; these structures involve the delocalization of pi electrons, which can occupy multiple positions within the molecule.
- The actual structure of a molecule is called a resonance hybrid, which is the most stable form and has the lowest energy compared to individual resonance structures.
- For the carbonate ion (CO3^2-), the bond order is calculated as 1.33, with the charge distributed equally among the three resonance structures, leading to an average bond order.
- Bond length is defined as the internuclear distance between two atoms, which is influenced by factors such as atomic size, bond order, and electronegativity difference; larger atoms result in longer bond lengths.
- The bond energy is the energy required to break one mole of a bond, and it is directly related to bond order; higher bond orders correspond to higher bond energies and shorter bond lengths.
- The bond angle is determined by the hybridization of the central atom; for example, sp3 hybridization results in a bond angle of 109.5°, sp2 gives 120°, and sp gives 180°.
- Factors affecting bond angles include the hybridization of the central atom, the number of lone pairs, and the electronegativity of the central atom; larger terminal atoms increase bond angles due to greater repulsion.
- Polar bonds occur between atoms with different electronegativities, leading to an uneven distribution of electron density, while non-polar bonds occur between identical atoms; the ionic character of a bond can be estimated using the formula 16 * ΔEN + 3.5 * (ΔEN)^2, where ΔEN is the difference in electronegativity.
52:13
Understanding Damping Moments and Molecular Polarity
- The damping moment, denoted as "u," involves two atoms (a and b) with partial charges, where the positive charge is labeled as positive partial and the negative charge as negative partial. The internuclear distance is represented by "d," and the damping moment is calculated using the formula: damping moment = q * d, where "q" is the charge and "d" is the distance.
- The damping moment is a vector quantity, meaning it has both magnitude and direction. The direction of the moment is determined by the electronegativity of the atoms involved; the more electronegative atom will attract the moment towards itself.
- To determine if a bond is polar or non-polar, one must calculate the dipole moments of all bonds in the molecule and find their vector sum. For example, in carbon dioxide (CO2), the dipole moments from the oxygen atoms cancel each other out, resulting in a net dipole moment of zero, indicating that CO2 is a non-polar molecule.
- In the case of boron trifluoride (BF3), the dipole moments from the fluorine atoms also cancel out due to their symmetrical arrangement, leading to a net dipole moment of zero, thus classifying BF3 as a non-polar molecule.
- Regular geometry refers to a molecular structure where the central atom has no lone pairs and all surrounding atoms are identical. In such cases, the net dipole moment is zero, indicating non-polarity.
- To calculate the percentage of ionic character in a covalent bond, the formula used is: (observed dipole moment / calculated dipole moment) * 100%. This requires both the observed dipole moment and the calculated dipole moment to be provided in the problem.
- Back bonding occurs when an atom with a vacant orbital interacts with another atom that has a lone pair, allowing for the overlap of orbitals. For example, in BF3, the vacant p-orbital of boron overlaps with the lone pair of fluorine, facilitating back bonding.
- The presence of back bonding increases the bond order, strengthens the bond, and decreases the bond length. For instance, BF3 exhibits significant back bonding due to the effective overlap of 2p orbitals, enhancing its stability.
- Bridge bonding is characterized by the formation of a bond between two atoms through a third atom, often seen in compounds like B2H6, where hydrogen acts as a bridge between two boron atoms. This type of bond can be classified as either electron-deficient or coordinate bridge bonding.
- Molecular orbital theory explains bonding through the combination of atomic orbitals to form molecular orbitals, which can accommodate electrons from the participating atoms, providing a more comprehensive understanding of molecular structure and behavior.
01:06:15
Understanding Molecular Orbitals and Bonding
- Molecular orbitals are formed through the combination of atomic orbitals, where two atomic orbitals combine to create two molecular orbitals, and four atomic orbitals can create four molecular orbitals.
- The energy levels of molecular orbitals differ, with bonding molecular orbitals having lower energy and greater stability compared to antibonding molecular orbitals, which have higher energy and less stability.
- Constructive interference occurs when atomic orbitals overlap in the same phase, resulting in bonding molecular orbitals, while destructive interference leads to antibonding molecular orbitals.
- Bonding molecular orbitals may contain nodes, but antibonding molecular orbitals always contain nodes, indicating regions of zero electron density.
- The types of molecular orbitals studied include sigma (σ) and pi (π) orbitals, with sigma orbitals formed from head-on overlaps and pi orbitals formed from sidewise overlaps.
- The sequence of molecular orbital energy levels is crucial, with the order being sigma 1s, sigma star 1s, sigma 2s, sigma star 2s, and then pi 2p orbitals, depending on whether sp mixing occurs.
- The filling of electrons in molecular orbitals follows three rules: the Pauli Exclusion Principle, Hund's Rule, and the Aufbau Principle, which dictate how electrons occupy the orbitals.
- The bond order of a molecule can be calculated using the formula: bond order = (number of bonding electrons - number of antibonding electrons) / 2, with a bond order of zero or less indicating that the molecule is unstable.
- Paramagnetism occurs in molecules with unpaired electrons, while diamagnetism occurs in molecules with all paired electrons; for example, O2 is paramagnetic due to its unpaired electrons.
- Fajan's Rule explains the covalent character in ionic bonds, stating that the polarizing power of cations increases with smaller size and higher charge, while the polarizability of anions increases with larger size, affecting the bond's character.
01:20:45
Understanding Molecular Interactions and Bonding Strengths
- Polarizing power is significant when the percentage of polar molecules is high, indicating a strong interaction between them, as discussed in the context of Kovalenko's principles.
- The Van der Waals forces are categorized as weak forces, with the first type being the dipole-dipole attraction, which occurs between polar molecules where the positive end of one molecule attracts the negative end of another.
- Induced dipole interactions occur when a polar molecule induces a dipole in a non-polar molecule, leading to an attraction that depends on the polarizability of the non-polar molecule's electron cloud.
- The strength of the induced dipole attraction is influenced by the polarizability of the non-polar molecule, which is determined by the ease of distortion of its electron cloud.
- London dispersion forces, also known as dispersion forces, are a type of Van der Waals force that increases with the size and surface area of the molecules involved, making larger molecules exhibit stronger attractions.
- Hydrogen bonds are a specific type of attraction that occurs when hydrogen is bonded to highly electronegative elements like fluorine, oxygen, or nitrogen, creating a partial positive charge on hydrogen and a partial negative charge on the electronegative atom.
- For a hydrogen bond to form, the electronegative atom must have a small size and a lone pair of electrons available to interact with the hydrogen atom from another molecule.
- There are two types of hydrogen bonds: intermolecular hydrogen bonds, which occur between different molecules (e.g., HF and H2O), and intramolecular hydrogen bonds, which occur within the same molecule (e.g., in salicylaldehyde).
- The strength of hydrogen bonds is influenced by the electronegativity of the atoms involved; a higher electronegativity leads to stronger hydrogen bonds, while the Lewis basicity of the atoms can also affect bond strength.
- The presence of hydrogen bonding significantly impacts the physical properties of substances, such as boiling points, where substances with stronger hydrogen bonds (like H2O) have higher boiling points compared to those with weaker interactions (like H2S).
01:35:16
Hydrogen Bonding and Solubility Explained
- The text discusses the relationship between vapor pressure and hydrogen bonding, stating that higher vapor pressure correlates with stronger hydrogen bonds, resulting in less vapor formation. This indicates that solubility in water is inversely proportional to the strength of intermolecular hydrogen bonds.
- Alcohol is highlighted as a soluble organic substance in water due to its ability to form hydrogen bonds with water molecules, which facilitates its dissolution through the breaking of hydrogen bonds.
- The concept of hydration energy or solvation energy is introduced, explaining that when a solid dissolves in water, energy is released due to the attraction between water molecules and the solute, specifically sodium ions (Na⁺), which is termed hydration energy.
- Hydration energy is directly proportional to the charge of the ion; higher charges lead to stronger attractions and more energy release during hydration. Conversely, hydration energy is inversely proportional to the size of the ion, meaning larger ions have lower hydration energy.
- The text explains that lattice enthalpy and hydration enthalpy are crucial for solubility; for a substance to be soluble in water, its lattice enthalpy must be lower than its hydration enthalpy, facilitating the dissolution process.
- Bent's rule is mentioned, which states that more electronegative elements occupy orbitals with lower s character, affecting hybridization and bond angles in molecules like NH₃, where nitrogen has a lone pair that influences its hybridization.
- Dragon's rule is introduced, indicating that if the central atom in a molecule is from the third period or below and the terminal atom's electronegativity is less than 2.5, the bond angles will approximate 90 degrees due to the use of pure p orbitals instead of hybridized orbitals.
- The text concludes with a brief overview of metallic bonding, describing it as the attraction between metal ions and a sea of delocalized electrons, which contributes to the hardness of metals, with stronger metallic bonds resulting in harder materials.
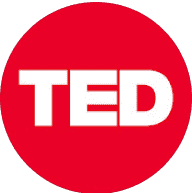
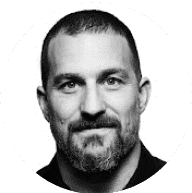
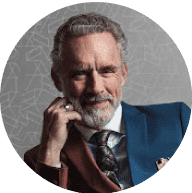

