Chapter 3: Prokaryotic Cells
Dr. Julie Wells・2 minutes read
Prokaryotic and eukaryotic cells differ in structures like having circular vs. linear chromosomes and membrane-bound organelles, showcasing unique characteristics and functions. Bacteria exhibit various shapes, movement patterns, and structures, with biofilms providing advantages like protection and resistance to antibiotics, emphasizing the importance of understanding cell anatomy and functions.
Insights
- Prokaryotic cells lack a membrane-bound nucleus, while eukaryotic cells have paired linear chromosomes enclosed within a nuclear membrane.
- Eukaryotic cells possess membrane-bound organelles, unlike prokaryotic cells, and may have cell walls made of polysaccharides like chitin or cellulose.
- Bacteria exhibit various shapes like rod-shaped (bacillus), spherical (coccus), and spiral forms (vibrio, spirillum, spirochete) with distinct morphologies.
- Biofilms, complex microbial communities, offer bacteria advantages like shared nutrients, protection, and resistance to antibiotics, aiding in conjugation for DNA transfer.
- Gram-positive bacteria have a thick peptidoglycan layer, while gram-negative bacteria have a thin layer and an outer membrane with lipopolysaccharides.
- Passive transport moves molecules down concentration gradients without energy, while active transport requires energy like ATP to move molecules against the gradient, with examples like ion pumps and cotransporters.
Get key ideas from YouTube videos. It’s free
Recent questions
What are the basic shapes of prokaryotic cells?
Rod-shaped, spherical, spiral forms with distinct morphologies.
Related videos
Summary
00:00
Cell Anatomy: Prokaryotic vs Eukaryotic Cells
- The video covers the functional anatomy of prokaryotic cells in chapter three, with eukaryotic cells to be discussed in the next unit.
- A white blood cell was shown chasing a bacterium, highlighting the abilities of cells like movement, recognition, and engulfing foreign invaders.
- All cells share common structures: a plasma or cell membrane, chromosomes carrying genes, ribosomes for protein synthesis, and cytosol as the cell's fluid.
- Prokaryotic cells lack a membrane-bound nucleus, with one or few circular chromosomes not enclosed in a membrane, unlike eukaryotic cells with paired linear chromosomes within a nuclear membrane.
- Prokaryotic cells lack histones, while eukaryotic cells have them to facilitate packing of long DNA sequences.
- Eukaryotic cells possess membrane-bound organelles, unlike prokaryotic cells, and may have cell walls made of polysaccharides like chitin or cellulose.
- Prokaryotic cells typically have peptidoglycan in their cell walls, while archaea cells may have pseudomurin, distinct from peptidoglycan.
- Prokaryotic cells reproduce via binary fission, while eukaryotic cells use the mitotic spindle for replication.
- Bacteria are typically monomorphic, maintaining one shape, while some are pleomorphic, able to change shape due to environmental factors.
- Basic shapes of prokaryotic cells include rod-shaped (bacillus), spherical (coccus), and spiral forms like vibrio, spirillum, and spirochete, each with distinct morphologies.
19:48
Diverse Bacterial Shapes and Structures Explained
- Bacillus bacteria can be shaped like a bacillus, but not all bacillus-shaped bacteria belong to the genus Bacillus.
- A star-shaped bacteria was discovered in the tundra, belonging to the genus Stella, showcasing unique morphology.
- Bacteria can have various shapes like rectangular, resembling plant cells, with some falling under the genus Haloarcula.
- Bacterial arrangements include pairs (diplo), clusters (staphylo), and chains (strepto), based on groupings.
- Bacillus-shaped bacteria do not form clusters due to their reproduction method along the horizontal axis.
- Prokaryotic cell structures include common elements like cell membrane, chromosome, ribosomes, and cytoplasm.
- Some bacteria possess additional structures like S layer, fimbriae, outer membrane, and cell wall, not universal.
- External structures of bacteria include appendages like flagella, pili, and fimbriae for motility and attachment.
- Glycocalyx, a gelatinous layer, can form slime or capsules, aiding in adherence, biofilm formation, and virulence.
- Biofilms, complex microbial communities, offer bacteria advantages like shared nutrients, protection from harmful factors, and resistance to antibiotics.
39:18
Biofilms: Antibiotic resistance, DNA transfer, deadly infections
- Bacteria in biofilms are more resistant to antibiotics and the body's immune system due to the protective slime layer.
- Biofilms aid in conjugation, facilitating DNA transfer between bacteria in close proximity.
- Streptococcus pneumoniae causes pneumonia, while Klebsiella pneumoniae can lead to pneumonia or UTIs if it leaves the gut.
- Hemophilus influenzae causes meningitis, not influenza, and Pseudomonas aeruginosa is fatal in burn victims due to its resistance to antibiotics and disinfectants.
- Pseudomonas can also infect cystic fibrosis patients, leading to fatal lung infections.
- Neisseria meningitidis causes meningitis and is transmitted through saliva, posing a fatal risk.
- Cryptococcus neoformans, a yeast found in bird droppings, can be fatal for immunocompromised individuals like AIDS patients.
- The bacterial glycocalyx aids in biofilm formation, surface adhesion, and protection from dehydration and nutrient loss.
- An S layer, a single protein layer, is produced by bacteria in hostile environments.
- Bacterial motility is primarily achieved through flagella, consisting of a filament, hook, and basal body, with different arrangements dictating movement patterns.
57:40
"Bacterial Movement and Genetic Functions Explained"
- Bacteria exhibit movement in response to stimuli like attractants, with chemotaxis referring to movement in response to a chemical stimulus, leading to positive or negative chemotaxis.
- Phototaxis involves movement in response to light, with plants displaying positive phototaxis to grow towards sunlight for photosynthesis.
- Bacteria move using flagella, with flagellar proteins known as h antigens aiding in determining bacterial strains based on the antigens present.
- E. coli strains are identified by specific antigens like o157 h7, which can cause food poisoning due to the shiga toxin gene acquired from Shigella bacteria.
- Endoflagella, also known as axial filaments, are flagella attached to the outside of the cell, found in spirochetes like Treponema pallidum causing syphilis.
- Fimbriae are proteinaceous structures allowing bacteria to attach to surfaces, like E. coli attaching to intestinal microvilli for colonization.
- Pili, exclusive to gram-negative cells, aid in conjugation for genetic material transfer, attachment, and motility, assisting in bacterial functions.
- The cytoplasm in prokaryotes serves as a pool for cell synthesis building blocks and energy sources, with the nucleoid region containing bacterial chromosomes and plasmids.
- Bacterial chromosomes carry essential genes, while plasmids carry non-crucial genes, providing advantages like antibiotic resistance, replicating independently of cell division.
- Prokaryotic ribosomes, composed of ribosomal RNA and proteins, aid in protein synthesis, with small 30s and large 50s subunits collectively forming 70s ribosomes.
01:17:48
Cell Inclusions and Reserves in Bacteria
- A 60s and a 40 combined weigh 80s, while a 30s and a 50s combined give 70s.
- Eukaryotic ribosomes consist of a 60s and a 40s.
- Inclusions in cells serve as temporary reserve deposits, storing various substances.
- Metachromatic granules store phosphate reserves for energy production.
- Polysaccharide granules store energy reserves in the form of sugars.
- Lipid inclusions and sulfur granules also serve as energy reserves.
- Carboxysomes store the enzyme rubisco for carbon dioxide fixation during photosynthesis.
- Gas vacuoles help bacteria float, aiding in accessing sunlight for photosynthesis.
- Magnetosomes contain iron oxide inclusions to neutralize toxic hydrogen peroxide.
- Endospores, found mainly in gram-positive bacteria like Clostridium and Bacillus, are structures for survival in adverse conditions, with longevity up to millions of years.
01:37:13
"Bacterial Cell Structures and Gram Reactions"
- Capsule is a structure protecting against phagocytosis, unlike endospore which responds to harsh conditions.
- Flagellum and axial filaments aid in motility, while ribosomes synthesize proteins.
- Bacterial cell walls prevent osmotic lysis and contribute to pathogenicity, primarily made of peptidoglycan.
- Peptidoglycan consists of nag and nam disaccharides, forming a polymer repeating in rows.
- Carbohydrate backbones in peptidoglycan are linked by peptide cross bridges with alternating d and l amino acids.
- Gram-positive cell walls have thick peptidoglycan with teichoic acids, while gram-negative have a thin layer and lack teichoic acids.
- Teichoic acids in gram-positive cells regulate cation movement and have lipoteichoic and wall teichoic acids.
- Gram-negative cell walls have an outer membrane with lipopolysaccharides, protecting against phagocytosis and complement.
- Lipopolysaccharides in gram-negative bacteria consist of o polysaccharide, core polysaccharide, and lipid a, with lipid a containing endotoxin.
- Knowing the gram reaction of an infection is crucial for antibiotic selection, as penicillin targets gram-positive thick peptidoglycan, while bacteriostatic drugs inhibit bacterial growth in gram-negative infections.
01:57:47
Understanding Bacterial Cell Membranes and Walls
- The yellow color in the images represents the cell membrane, present in all cells.
- Gram-positive bacteria have a thicker peptidoglycan layer compared to gram-negative bacteria, which have a thinner layer.
- Gram-negative bacteria possess an additional outer membrane absent in gram-positive bacteria.
- The periplasmic space is the area between the peptidoglycan and the outer membrane in gram-negative bacteria.
- Gram-positive bacteria are not typically thought to have a periplasmic space, with any observed gap likely due to procedural artifacts.
- The Gram stain procedure involves four steps: crystal violet staining, iodine addition, decolorization, and safranin counterstaining.
- Gram-positive bacteria retain crystal violet and appear purple, while gram-negative bacteria lose crystal violet and stain pink with safranin.
- Most bacteria are categorized as either gram-positive or gram-negative, with some being gram-variable, like Bacillus and Clostridium.
- Atypical cell walls, such as those of Mycobacterium tuberculosis and Mycoplasma pneumoniae, exhibit unique characteristics and resistance to treatments.
- Enzymes like lysozyme and chemicals like penicillin can damage bacterial cell walls by targeting peptidoglycan components.
02:18:07
Bacterial Cell Structures and Functions
- Gram-positive bacteria are more affected by certain chemicals due to their thick layer of peptidoglycan.
- Streptococcus pyogenes, a gram-positive bacteria causing strep throat, has adaptations to resist lysozyme.
- Lysozyme typically inhibits peptidoglycan, affecting gram-positive bacteria more than gram-negatives.
- The outer membrane of gram-negative bacteria contains lipopolysaccharide.
- Sterols are found in mycoplasma due to their lack of a cell wall.
- Mycolic acid is found in mycobacterium bacteria.
- Teichoic acids are exclusive to gram-positive bacteria.
- The cell membrane is a fluid mosaic model with proteins embedded in it.
- Phospholipid bilayer in the cell membrane has hydrophilic heads and hydrophobic tails.
- Prokaryotic cells synthesize ATP in their cell membrane, lacking membrane-bound organelles like mitochondria.
02:36:01
Cellular Transport and Membrane Permeability
- Cells maintain shape through attachment to the cytoskeleton and extracellular matrix.
- Traffic across the membrane is crucial for cell survival, allowing intake of essential nutrients and removal of waste products.
- Cells need to take in molecules for energy and expel metabolic waste products like carbon dioxide.
- Movement of ions like sodium, potassium, and calcium is vital for cellular functions.
- Phospholipid bilayer structure consists of hydrophilic heads and hydrophobic tails, affecting molecule permeability.
- Hydrophobic molecules like steroids and small hydrocarbons can freely cross the membrane.
- Hydrophilic molecules like ions and glucose cannot cross the hydrophobic core of the membrane on their own.
- Diffusion involves molecules moving from high to low concentration spontaneously, dependent on thermal motion.
- Diffusion is faster at warmer temperatures due to increased molecular motion.
- Water, being polar, moves down its concentration gradient, interacting with solutes like salt through hydration cells.
02:52:53
Cellular Water Movement and Passive Transport Overview
- Water moves from high concentration to low concentration in osmosis.
- Tonicity determines if a cell gains or loses water, comparing solute concentrations.
- Hypertonic solutions have higher solute concentrations, causing water to move out of cells.
- In animal cells, water loss from hypertonic solutions leads to cell shrinkage, while in plant cells, plasmolysis occurs.
- Hypotonic solutions have lower solute concentrations, causing water to move into cells.
- In animal cells, water influx from hypotonic solutions leads to cell bursting, while in plant cells, turgor pressure increases.
- Isotonic solutions have equal solute concentrations, resulting in balanced water movement in and out of cells.
- Drinking ocean water leads to dehydration due to the hypertonic environment created outside cells.
- Passive transport moves molecules down concentration gradients without energy.
- Simple diffusion and facilitated diffusion are types of passive transport, with the latter requiring transport proteins for molecules unable to cross membranes independently.
03:09:50
Cell Transport Mechanisms and Disorders Explained
- Carrier proteins are transmembrane proteins that change shape upon binding to solute, allowing the solute to enter the cell.
- Passive transport moves molecules from high to low concentration without requiring energy, unlike active transport.
- Active transport involves transport proteins and requires energy, typically in the form of ATP, to move molecules against the concentration gradient.
- Examples of active transport include ion pumps like the proton pump, which moves protons against the gradient, requiring ATP.
- Ion pumps like the proton pump are electrogenic, creating a charge difference across the membrane.
- Cotransporters use two transmembrane proteins to move molecules up and down the concentration gradient simultaneously.
- Bulk transport methods include exocytosis, where vesicles fuse with the plasma membrane to release contents outside the cell.
- Endocytosis, on the other hand, involves getting large molecules or particles into the cell, with types like phagocytosis and pinocytosis.
- Receptor-mediated endocytosis is a specific type of endocytosis where receptors bind to ligands for targeted molecule uptake.
- Familial hypercholesterolemia, a genetic disorder with defective LDL receptors, leads to high cholesterol levels in the blood, potentially causing heart disease if untreated.
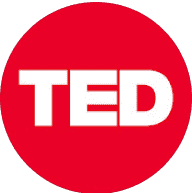
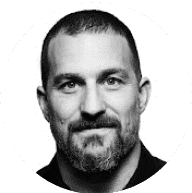
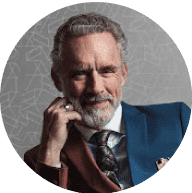

