Chapter 18 Regulation of Gene Expression
Jill Barker・30 minutes read
Gene expression regulation in prokaryotes and eukaryotes is shaped by environmental factors, with prokaryotes utilizing operons for transcription control and eukaryotes relying on chromatin modifications and transcription factors. The significance of these mechanisms is underscored by their roles in cellular differentiation, embryonic development, and cancer progression, where mutations can lead to unchecked cell growth.
Insights
- Gene expression in prokaryotes and eukaryotes is intricately regulated by various mechanisms, with prokaryotes utilizing operons to control gene activity through repressors and inducers, while eukaryotes employ complex processes such as chromatin modifications and transcription factors that allow for precise control of individual genes, emphasizing the differences in regulatory strategies between these two domains of life.
- The regulation of gene expression is critical in developmental biology and disease, as seen in the role of maternal cytoplasmic determinants in embryonic differentiation and the impact of mutations in proto-oncogenes and tumor suppressor genes on cancer progression, highlighting how gene regulation is essential not only for normal cellular functions but also for preventing uncontrolled growth and maintaining cellular integrity.
Get key ideas from YouTube videos. It’s free
Recent questions
What is gene expression regulation?
Gene expression regulation refers to the processes that control the timing and amount of gene expression in cells. This regulation is crucial for ensuring that genes are expressed at the right times and in the right amounts, which is essential for proper cellular function and development. In prokaryotes, gene expression is often regulated through operons, which are clusters of genes controlled by a single promoter. In contrast, eukaryotic gene expression involves more complex mechanisms, including chromatin modifications, transcription factors, and post-transcriptional processes. These regulatory mechanisms allow cells to respond to environmental changes and developmental cues, ensuring that the right proteins are produced when needed.
How do operons function in bacteria?
Operons are a fundamental feature of bacterial gene regulation, consisting of a group of related genes that are transcribed together under the control of a single promoter. This arrangement allows bacteria to efficiently coordinate the expression of genes that are involved in related functions, such as metabolic pathways. The operon can be turned on or off by regulatory proteins that interact with specific DNA sequences. For instance, a repressor protein can bind to the operator region of the operon, blocking RNA polymerase from transcribing the genes when the repressor is active. Conversely, in the presence of an inducer, the repressor can be inactivated, allowing transcription to proceed. This mechanism enables bacteria to adapt quickly to changes in their environment by regulating gene expression in response to available nutrients.
What is the role of transcription factors in eukaryotes?
Transcription factors play a critical role in the regulation of gene expression in eukaryotic cells. These proteins bind to specific DNA sequences known as control elements, which are located upstream of the gene's promoter. By interacting with RNA polymerase and other components of the transcription machinery, transcription factors can enhance or inhibit the transcription of target genes. This regulation is essential for the precise control of gene expression, allowing cells to respond to developmental signals and environmental changes. Additionally, transcription factors can influence chromatin structure, making DNA more or less accessible for transcription. The complex interplay between transcription factors and chromatin modifications is key to the regulation of gene expression in multicellular organisms.
What is post-transcriptional regulation?
Post-transcriptional regulation refers to the processes that occur after the transcription of DNA into RNA, influencing the stability, processing, and translation of mRNA. This regulation is crucial for determining the final levels of protein synthesis in a cell. One key aspect of post-transcriptional regulation is mRNA splicing, where introns are removed and exons are joined together, allowing for the production of different mRNA variants from a single gene. Additionally, the stability of mRNA in the cytoplasm can be regulated by sequences at the 3' end, which determine how long the mRNA persists before degradation. Regulatory proteins can also bind to mRNA to inhibit translation, ensuring that proteins are synthesized only when needed. This layer of regulation allows for fine-tuning of gene expression in response to cellular conditions.
How do mutations affect cancer development?
Mutations play a significant role in the development of cancer by disrupting the normal regulation of cell growth and division. Cancer typically arises from mutations in proto-oncogenes, which promote cell division, and tumor suppressor genes, which normally inhibit excessive cell growth. When proto-oncogenes mutate into oncogenes, they can lead to uncontrolled cell proliferation. Similarly, mutations in tumor suppressor genes, such as p53, can prevent the necessary checkpoints in the cell cycle from functioning, allowing damaged cells to continue dividing. The development of cancer usually requires multiple mutations, including at least one mutation that activates an oncogene and several that inactivate tumor suppressor genes. As individuals age, the cumulative genetic damage increases the risk of cancer, highlighting the importance of gene regulation in maintaining cellular integrity and preventing tumorigenesis.
Related videos
NCERT Wallah
Molecular Basis of Inheritance 08 | Human Genome Project, Lac Operon | Class 12th/CUET
J. Christopher Anderson
Constitutive Promoters
Eric Sweet
Central Dogma YT
Anna Freud
Epigenetics and Mental Health: A brief guide to the research | UK Trauma Council
Parth Momaya
Heredity and Evolution SSC Class 10 ONE SHOT || Parth Momaya || Maharashtra State Board
Summary
00:00
Gene Expression Regulation in Prokaryotes and Eukaryotes
- Gene expression regulation in prokaryotes and eukaryotes is influenced by environmental factors, crucial for multicellular eukaryotic development and cellular differentiation.
- In bacteria, gene expression is controlled by operons, which are groups of related genes regulated by a single on/off switch located in the promoter region.
- Operons can be repressed by a protein repressor that binds to the operator, preventing RNA polymerase from transcribing the genes when active.
- Repressible operons, like the trp operon in E. coli, remain active until sufficient tryptophan binds to the repressor, changing its shape and shutting down transcription.
- Inducible operons, such as the lac operon, are typically off but can be activated when an inducer, like allolactose, binds to the repressor, allowing transcription to occur.
- Catabolic pathways typically involve inducible enzymes, while anabolic pathways involve repressible enzymes, with operons being subject to negative control mechanisms.
- Positive control is exemplified by the catabolic activator protein (CAP), which enhances RNA polymerase binding to the lac operon when cAMP levels are high due to low glucose.
- Eukaryotic gene expression regulation involves chromatin modifications, RNA processing, and mRNA degradation, impacting protein synthesis and cellular function.
- Chromatin structure can be modified through acetylation, methylation, and phosphorylation, influencing gene accessibility for transcription without altering DNA sequences.
- Transcription factors bind to control elements upstream of the transcription start point, determining gene expression levels by interacting with RNA polymerase and chromatin structure.
19:39
Eukaryotic Gene Expression and Regulation Insights
- Eukaryotic gene expression involves mediator proteins and transcription factors that prepare DNA for RNA polymerase binding, differing from prokaryotic operons which express genes simultaneously.
- Each eukaryotic gene has its own promoter and control elements, often located on different chromosomes, but they share similar control elements for coordinated transcription.
- Chromatin loops from different chromosomes can cluster in specific nuclear locations rich in transcription factors and RNA polymerase, facilitating simultaneous transcription of related genes.
- Post-transcriptional regulation includes splicing, where different mRNA molecules are produced from the same primary transcript, allowing for varied combinations of exons and introns.
- Eukaryotic mRNA has sequences at the 3' end that determine its lifespan in the cytoplasm, preventing indefinite persistence before degradation.
- Regulatory proteins can bind to mRNA to inhibit translation, ensuring that necessary enzymes for processes like fertilization are available simultaneously for cell division.
- Proteins tagged with ubiquitin are targeted for degradation by proteasomes, recycling amino acids for new protein synthesis when they are no longer needed.
- Non-coding RNAs, such as microRNAs and small interfering RNAs, regulate gene expression by binding to mRNA, preventing translation, or degrading unwanted mRNA.
- Maternal cytoplasmic determinants influence early embryonic development, affecting gene expression and differentiation as the fertilized egg divides into various cell types.
- Cancer can arise from mutations in proto-oncogenes and tumor suppressor genes, leading to uncontrolled cell growth and division, highlighting the importance of gene regulation in cell cycle control.
39:53
Mutations Driving Cancer Development Explained
- Mutations in the ras proto-oncogene can lead to hyperactive ras proteins, causing excessive cell division, while p53 mutations prevent the necessary cessation of the cell cycle, allowing damaged DNA to propagate.
- Cancer development typically requires multiple mutations, including at least one proto-oncogene converting to an oncogene and several tumor suppressor gene mutations, with age increasing cancer risk due to cumulative genetic damage.
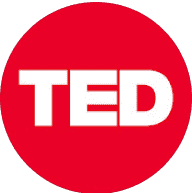
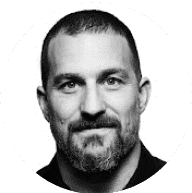
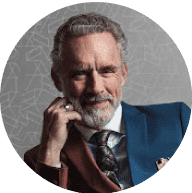

