Cell Biology | Passive & Active Transport | Endocytosis & Exocytosis
Ninja Nerd・69 minutes read
Simple diffusion allows nonpolar molecules like oxygen and carbon dioxide to move across the cell membrane without energy, while facilitated diffusion employs transport proteins for larger or charged molecules. Understanding these transport mechanisms is essential for grasping cellular functions and their implications in health, including the role of active transport like the sodium-potassium ATPase in maintaining ion balance.
Insights
- Simple diffusion is a key process that allows nonpolar molecules, such as oxygen and carbon dioxide, to move freely across cell membranes from areas of high concentration to low concentration without the need for energy, highlighting the importance of molecular properties in cellular transport.
- The structure of the cell membrane, composed of phospholipids with polar heads and nonpolar tails, selectively permits the passage of nonpolar substances while blocking charged molecules, underscoring the significance of membrane composition in regulating what enters and exits the cell.
- Facilitated diffusion and active transport mechanisms, such as the sodium-potassium ATPase and various ion channels, are essential for maintaining cellular function by enabling the movement of larger or charged molecules against their concentration gradients, illustrating the complexity of cellular transport systems.
- Understanding transport mechanisms, including vesicular transport methods like endocytosis and exocytosis, is critical for recognizing their roles in health and disease, as seen in conditions like familial hypercholesterolemia and the effects of medications that target these processes.
Get key ideas from YouTube videos. It’s free
Recent questions
What is simple diffusion?
Simple diffusion is a passive transport process that allows molecules to move from an area of high concentration to an area of low concentration without the use of energy (ATP). This mechanism is crucial for the movement of small, nonpolar molecules, such as oxygen and carbon dioxide, across cell membranes. The cell membrane, composed of phospholipids, has a structure that permits nonpolar substances to diffuse freely while restricting charged molecules. The efficiency of simple diffusion is influenced by factors such as the concentration gradient, surface area, and membrane thickness, making it a fundamental process for maintaining cellular homeostasis and facilitating gas exchange in living organisms.
How do molecules cross cell membranes?
Molecules cross cell membranes primarily through processes like simple diffusion and facilitated diffusion. Simple diffusion allows nonpolar molecules, such as oxygen and carbon dioxide, to move freely across the lipid bilayer of the cell membrane due to their compatibility with the nonpolar fatty acid tails of phospholipids. In contrast, facilitated diffusion involves specific transport proteins that assist larger or charged molecules in crossing the membrane without the expenditure of energy. This process is essential for the transport of substances like glucose and ions, ensuring that cells can maintain their internal environment and respond to changes in their surroundings effectively.
What is osmosis in biology?
Osmosis is a specific type of facilitated diffusion that refers to the movement of water molecules across a selectively permeable membrane from an area of high water concentration to an area of low water concentration. This process is driven by the concentration of solutes, such as sodium or glucose, on either side of the membrane. Osmosis is crucial for maintaining cellular turgor pressure and overall fluid balance within organisms. Specialized proteins called aquaporins facilitate the rapid movement of water, allowing cells to respond to osmotic changes efficiently. Understanding osmosis is vital for grasping how cells regulate their internal environments and maintain homeostasis.
What are ion channels?
Ion channels are specialized protein structures embedded in cell membranes that facilitate the movement of ions across the membrane. These channels can be classified into various types, including leaky channels, voltage-gated channels, ligand-gated channels, and mechanically gated channels. Each type serves a specific function; for instance, voltage-gated channels open in response to changes in membrane potential, allowing ions like sodium and calcium to flow into the cell, which is essential for generating action potentials in neurons and muscle cells. Understanding ion channels is critical for comprehending how electrical signals are transmitted in the body and how various physiological processes are regulated.
What is active transport in cells?
Active transport is a cellular process that moves substances against their concentration gradient, requiring energy in the form of ATP. This mechanism is essential for maintaining the proper balance of ions and nutrients within cells. A key example of active transport is the sodium-potassium ATPase, which pumps sodium ions out of the cell and potassium ions into the cell, crucial for maintaining the resting membrane potential in neurons and muscle cells. Active transport can be classified into primary active transport, which directly uses ATP, and secondary active transport, which relies on the movement of one molecule down its gradient to drive the transport of another molecule against its gradient. Understanding active transport is vital for grasping how cells regulate their internal environments and respond to external changes.
Related videos
Cararra
AP Biology Unit 2 Review: Cell Structure and Function
Amber Stokes
3020 Lecture 3
FuseSchool - Global Education
Transport In Cells: Active Transport | Cells | Biology | FuseSchool
Doctor Maria's Biology Channel
Anatomy and Physiology Chapter 3 Cells Part B
Osmosis from Elsevier
Endocytosis and exocytosis
Summary
00:00
Mechanisms of Molecular Movement Across Membranes
- Simple diffusion is a passive process that allows molecules to move from high to low concentration without using ATP or energy, facilitating movement across the cell membrane.
- Molecules that can move via simple diffusion include oxygen, carbon dioxide (CO2), steroid hormones (like testosterone and estrogen), and lipid-soluble drugs, all of which are nonpolar.
- The cell membrane consists of phospholipids with polar heads and nonpolar fatty acid tails, preventing charged molecules from crossing while allowing nonpolar substances to diffuse freely.
- The rate of diffusion for oxygen and CO2 is influenced by surface area, concentration gradient, membrane thickness, and molecular weight, with larger surface areas and higher gradients increasing diffusion rates.
- Facilitated diffusion is also a passive process, requiring no ATP, but it involves transport proteins (channels or carriers) to help molecules move across the cell membrane.
- Osmosis, a type of facilitated diffusion, describes water movement from high water concentration to low water concentration, influenced by solute concentrations like sodium or glucose.
- Aquaporins are specialized channel proteins that facilitate water movement across the cell membrane, allowing water to move according to concentration gradients.
- Different types of ion channels include leaky channels, voltage-gated channels, ligand-gated channels, and mechanically gated channels, each facilitating the movement of charged molecules.
- The concentration gradient remains a key factor in both simple and facilitated diffusion, with molecules moving from areas of high concentration to low concentration.
- Understanding these transport mechanisms is crucial for grasping how substances enter and exit cells, impacting cellular function and overall physiology.
16:30
Ion Transport Mechanisms Across Cell Membranes
- Charged molecules cannot move across the cell membrane due to the negative charge of phospholipids, necessitating specialized channels for their transport.
- Leaky potassium channels are crucial in neurons, allowing potassium to flow from high concentration inside the cell to low concentration outside, influencing resting membrane potential.
- Voltage-gated channels, such as sodium and calcium channels, open at a specific threshold (e.g., -55 millivolts), permitting ions to rush into the cell, essential for action potentials.
- Ligand-gated ion channels open when a specific molecule, like acetylcholine, binds, allowing sodium ions to flow into the cell, triggering action potentials and muscle contractions.
- Mechanically gated channels open in response to physical stress, such as pressure on pain receptors, allowing sodium ions to enter and induce action potentials signaling pain.
- Carrier-mediated facilitated diffusion involves transporters like GLUT4, which move glucose from high concentration outside the cell to low concentration inside, regulated by insulin.
- Facilitated diffusion is passive, requiring no ATP, and allows large or charged molecules to cross the cell membrane through specific protein channels or carriers.
- Primary active transport directly uses ATP to move substances against their concentration gradient, essential for maintaining cellular ion balance.
- The sodium-potassium ATPase is a key example of primary active transport, moving three sodium ions out and two potassium ions into the cell, requiring ATP for energy.
- Insulin and thyroid hormones can increase the activity of sodium-potassium ATPases, while drugs like digoxin inhibit this process, affecting cellular metabolism and ion balance.
32:20
Cellular Transport Mechanisms and Their Implications
- Digoxin increases heart contractility by inhibiting sodium-potassium ATPase, which is crucial for understanding cellular mechanisms in science and medicine.
- Calcium ATPases are essential for muscle relaxation, particularly in cardiac muscle, by transporting calcium ions from the cytoplasm into the sarcoplasmic reticulum.
- During muscle relaxation, calcium ions are pumped into the sarcoplasmic reticulum, requiring ATP to move calcium from low concentration in the cytoplasm to high concentration in the reticulum.
- Increased sympathetic nervous system activity raises norepinephrine and epinephrine levels, enhancing calcium ATPase activity and promoting more calcium storage in the sarcoplasmic reticulum.
- Proton pumps, specifically proton-potassium ATPases, in parietal cells of the stomach transport protons into the stomach lumen, requiring ATP to move protons against their concentration gradient.
- Proton pump inhibitors are medications that reduce hydrochloric acid production in the stomach, helping manage conditions like gastroesophageal reflux disease and peptic ulcer disease.
- Secondary active transport involves indirect ATP use, where one molecule moves down its concentration gradient while another moves against it, often utilizing sodium as the driving force.
- Sodium-glucose co-transporters (SGLT) allow glucose to enter cells against its concentration gradient by coupling its transport with sodium moving down its gradient.
- SGLT2 inhibitors are drugs used in diabetes management that prevent glucose reabsorption in the kidneys, leading to increased glucose excretion in urine and lower blood glucose levels.
- Understanding these transport mechanisms and their clinical implications is vital for applying basic science to medical practice, highlighting the connection between cellular processes and health outcomes.
47:52
Kidney Transport Mechanisms and Their Impacts
- The sodium-potassium-2-chloride symporter moves sodium, chloride, and potassium into kidney cells, with sodium and chloride moving down their gradients and potassium moving against its gradient.
- This symporter is crucial in the loop of Henle in kidneys, where it helps transport sodium, potassium, and chloride into the interstitial space, facilitating water reabsorption.
- Loop diuretics, like furosemide (Lasix), inhibit the sodium-potassium-2-chloride co-transporters, causing sodium, potassium, and chloride to accumulate in kidney tubules, pulling water into urine.
- The sodium-proton pump, an anti-porter in kidneys, moves sodium into cells while expelling protons, crucial for maintaining acid-base balance in the distal convoluted tubule.
- Increased aldosterone enhances sodium-proton pump activity, leading to more proton excretion and potentially causing metabolic alkalosis due to decreased blood proton levels.
- Conversely, low aldosterone reduces sodium-proton pump activity, resulting in less proton excretion, which can lead to metabolic acidosis from increased blood proton levels.
- The sodium-calcium exchanger, important in cardiac muscle, moves sodium into cells while expelling calcium, essential for regulating heart muscle contraction.
- Digoxin inhibits the sodium-potassium ATPase, causing sodium levels to drop outside the cell, preventing calcium from leaving and increasing calcium concentration, enhancing heart contractility.
- Vesicular transport includes endocytosis (cellular intake) and exocytosis (cellular expulsion), with endocytosis further divided into pinocytosis, phagocytosis, and receptor-mediated endocytosis.
- Pinocytosis, or "cellular drinking," involves the uptake of water and solutes into vesicles, requiring ATP for transport via motor proteins like kinesins and dyneins, especially in intestinal cells.
01:02:54
Cellular Mechanisms of Phagocytosis and Endocytosis
- Actin molecules assist in pulling pseudopods into the cell, forming a vesicle that contains pathogens, specifically bacteria, known as a phagosome during phagocytosis.
- The phagosome combines with an endosome, where protons are pumped in to create an acidic environment, essential for lysosomal enzyme activity, requiring ATP for this active transport.
- Lysosomes, containing hydrolytic enzymes, fuse with the acidified phagosome to form a phagolysosome, where bacterial components are broken down into smaller molecules.
- The breakdown process results in a secondary lysosome, which contains lysosomal enzymes and residual bacterial fragments, ready for exocytosis to expel waste from the cell.
- Exocytosis involves fusing the secondary lysosome with the cell membrane to release digested bacterial pieces into the lymphatic system, activating immune responses.
- Receptor-mediated endocytosis primarily occurs in liver cells, where low-density lipoprotein (LDL) binds to LDL receptors, initiating the uptake process.
- Clathrin proteins form a coated pit around the LDL-receptor complex, invaginating the membrane to create a vesicle that contains LDL and its receptors.
- Proton pumps in the endosome acidify the environment, weakening the bond between LDL and its receptors, leading to the separation of these components into distinct vesicles.
- The vesicle containing LDL receptors returns to the cell membrane to recycle receptors, while the LDL-containing vesicle fuses with lysosomes for breakdown and nutrient release.
- Familial hypercholesterolemia arises from defects in receptor-mediated endocytosis, leading to elevated LDL levels in the blood, increasing cardiovascular disease risks.
01:17:57
Protein Synthesis and Transport Mechanisms Explained
- The rough endoplasmic reticulum (RER) translates mRNA into proteins, modifies them by adding sugar residues, and forms vesicles containing these proteins using COPII proteins for transport to the Golgi apparatus.
- The Golgi apparatus further modifies proteins through phosphorylation and glycosylation, then packages them into vesicles for secretion, which may contain neurotransmitters like acetylcholine or hormones like insulin.
- Vesicle transport to the cell membrane relies on microtubules and motor proteins (kinesins and dyneins) powered by ATP; vesicle fusion with the membrane involves calcium-dependent interactions between v-SNAREs and t-SNAREs.
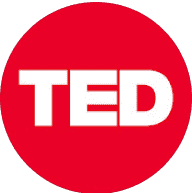
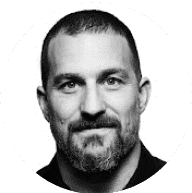
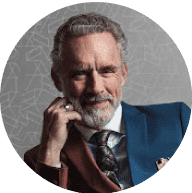

