Atomic Structure (full topic) | A Level
The Chemistry Tutor・59 minutes read
The chemical behavior of elements is determined by their atomic structure, specifically the arrangement of electrons around the nucleus, while fundamental particles like protons, neutrons, and electrons play vital roles in defining atomic properties and configurations. Additionally, concepts such as ionization energy trends, mass spectrometry for identifying elements, and the calculation of average atomic masses are crucial for understanding elements and their isotopes in relation to their configuration and abundance.
Insights
- The behavior and interactions of elements are determined by their atomic structure, particularly the arrangement of electrons around the nucleus, which is crucial for understanding their chemical properties and how they bond with other elements.
- Ionization energy, which is the energy required to remove electrons from atoms, varies across the periodic table and is influenced by factors such as atomic radius and nuclear charge, with significant jumps in energy levels indicating changes in electron configuration and group placement.
- Mass spectrometry provides a powerful tool for identifying elements and their isotopes by measuring the mass of atoms or molecules, revealing distinct peaks for different isotopes and allowing for precise calculations of relative atomic and molecular masses based on their unique mass values.
Get key ideas from YouTube videos. It’s free
Recent questions
What is an atomic number?
The atomic number is the number of protons in an atom's nucleus. It uniquely identifies an element and determines its position in the periodic table. Each element has a distinct atomic number, which also equals the number of electrons in a neutral atom, balancing the positive charge of protons with the negative charge of electrons. For example, carbon has an atomic number of 6, meaning it has 6 protons and 6 electrons. The atomic number is fundamental in understanding the chemical properties of an element, as it influences how the element interacts with others.
How do isotopes differ from each other?
Isotopes are variants of a particular chemical element that have the same number of protons but different numbers of neutrons in their nuclei. This difference in neutron count results in variations in atomic mass. For instance, carbon has isotopes such as carbon-12, carbon-13, and carbon-14, which have 6 protons but 6, 7, and 8 neutrons, respectively. While isotopes of an element exhibit similar chemical behavior due to their identical electron configurations, they can have different physical properties, such as stability and radioactivity. This distinction is crucial in fields like nuclear medicine and radiocarbon dating.
What is ionization energy?
Ionization energy is the amount of energy required to remove an electron from a gaseous atom or ion. It is a critical concept in chemistry, as it reflects the strength of the attraction between the nucleus and its electrons. The first ionization energy is the energy needed to remove the outermost electron, and this value typically increases with each subsequent electron removed due to the increasing positive charge of the ion, which attracts remaining electrons more strongly. Trends in ionization energy can indicate an element's position in the periodic table and its reactivity, with elements in the same group often exhibiting similar ionization energy patterns.
What is electron configuration?
Electron configuration describes the distribution of electrons in an atom's atomic orbitals. It provides insight into how electrons are arranged around the nucleus and is essential for understanding an element's chemical properties and reactivity. The configuration is typically written in a notation that indicates the energy levels and sublevels occupied by electrons, such as 1s² 2s² 2p⁶ for neon. The arrangement follows specific rules, including the Aufbau principle, which states that electrons fill lower energy orbitals first, and Hund's rule, which states that electrons will occupy degenerate orbitals singly before pairing up. This configuration influences how atoms bond and interact with one another.
What is a mass spectrometer used for?
A mass spectrometer is an analytical instrument used to measure the mass-to-charge ratio of ions, allowing for the identification and quantification of substances based on their unique mass values. The process involves ionizing chemical species, accelerating the ions, and then separating them based on their mass as they travel through a magnetic or electric field. The resulting mass spectrum displays peaks corresponding to different isotopes or molecular ions, providing valuable information about the composition and structure of the sample. Mass spectrometry is widely used in various fields, including chemistry, biochemistry, and environmental science, for applications such as determining molecular weights, analyzing complex mixtures, and identifying unknown compounds.
Related videos
Wacky Science
GENERAL CHEMISTRY explained in 19 Minutes
Wendy Riggs
Chem 4- Electrons
Cognito
GCSE Chemistry - Elements, Isotopes & Relative Atomic Mass #2
PassChem: Sponholtz Productions
Introduction to the Atom (English)
The Organic Chemistry Tutor
Intro to Chemistry, Basic Concepts - Periodic Table, Elements, Metric System & Unit Conversion
Summary
00:00
Atomic Structure and Electron Configuration Explained
- The chemical properties of elements depend on atomic structure, particularly the arrangement of electrons around the nucleus, which influences their behavior and interactions.
- Fundamental particles include protons, neutrons, and electrons, with protons and neutrons collectively called nucleons, held together by the strong nuclear force, significantly stronger than electrostatic forces.
- Protons have a mass of approximately 1.67 x 10^-27 kg, a charge of +1, while electrons have a negligible mass of 1/1840 of a proton and a charge of -1.
- The atomic number (Z) represents the number of protons in an atom, while the mass number (A) is the total number of nucleons (protons + neutrons) in the nucleus.
- For fluorine (atomic number 9), there are 9 protons and electrons, and with a mass number of 19, it has 10 neutrons (19 - 9 = 10).
- Isotopes have the same number of protons but different numbers of neutrons; for example, carbon isotopes have 6 protons but vary in neutron count (e.g., 6, 7, or 8 neutrons).
- Electron configuration describes how electrons are organized in shells; for carbon, the configuration is 2 in the first shell and 4 in the second (2, 4).
- The maximum number of electrons in energy levels follows the formula 2n^2; for example, the second level holds 8 electrons (2 x 2^2) and the third level can hold 18 electrons (2 x 3^2).
- Atomic orbitals, which define the regions where electrons are likely to be found, include s (spherical), p (three orientations), and d (five orientations), each with specific energy levels.
- Each atomic orbital can hold a maximum of 2 electrons; thus, the s sublevel holds 2, p holds 6, and d holds 10 electrons, contributing to the overall electron capacity of energy levels.
17:01
Understanding Electron Configuration and Ionization Energy
- Electrons exhibit a property called spin, represented by arrows: spin up (↑) for one direction and spin down (↓) for the opposite direction.
- When filling electron orbitals, always fill lower energy levels first for stability, following the order of 1s, 2s, and then 2p.
- Place electrons singly in orbitals before pairing them; for example, in oxygen, fill 2p with three single electrons before pairing the fourth.
- The electron configuration for lithium (3 electrons) is 1s² 2s¹, while sodium (11 electrons) is 1s² 2s² 2p⁶ 3s¹.
- For carbon (6 electrons), the configuration is 1s² 2s² 2p⁴, while argon (18 electrons) is 1s² 2s² 2p⁶ 3s² 3p⁶.
- Iron has 26 electrons, with the configuration 1s² 2s² 2p⁶ 3s² 3p⁶ 4s² 3d⁶, filling 4s before 3d.
- Transition metals fill 4s before 3d but often write 3d first; exceptions include chromium (3d⁵ 4s¹) and copper (3d¹⁰ 4s¹) for stability.
- Ionization energy is the energy needed to remove one mole of electrons from gaseous atoms, measured in kilojoules per mole.
- The first ionization energy is the lowest, increasing with each subsequent electron removed due to stronger positive charge attraction in ions.
- Successive ionization energies reveal group placement in the periodic table; large jumps indicate a change in energy levels, signaling outer electron count.
34:21
Ionization Energy Trends and Mass Spectrometry Insights
- Ionization energy trends in Period 3 show an overall increase, with a pattern of two increases, a dip, three increases, and another dip, indicating varying electron removal difficulty.
- The increase in ionization energy across Period 3 is due to increased nuclear charge, decreased atomic radius, and constant shielding, leading to stronger attraction between the nucleus and outer electrons.
- The first dip in ionization energy occurs when comparing elements with outer electrons in different subshells, specifically between 3s² and 3p¹, where 3p is easier to remove.
- The second dip is explained by electron pairing in the 3p subshell; 3p⁴ has paired electrons, making them easier to remove compared to the singly occupied 3p³.
- In Group 2, ionization energy decreases down the group due to increasing atomic radius and increased shielding, resulting in weaker attraction between the nucleus and outer electrons.
- A mass spectrometer measures the mass of atoms or molecules, providing precise relative atomic and molecular masses, essential for identifying elements based on their unique mass values.
- Relative atomic mass is calculated by comparing the average mass of an atom to 1/12 of a carbon-12 atom's mass, while relative molecular mass uses the same comparison for molecules.
- The time-of-flight mass spectrometer operates in four steps: ionization, acceleration, drift, and detection, with ions gaining kinetic energy and lighter ions traveling faster.
- Ionization can occur via an electron gun, where electrons knock off others from gaseous samples, or through an electron spray technique, where samples gain protons in a solvent.
- Mass spectra display isotopes as distinct peaks, with the heaviest peak indicating the molecular ion peak, essential for determining the molecular mass and identifying substances.
52:43
Understanding Mass Spectrometry and Chlorine Isotopes
- The mass spectrum generated by the electron spray mass spectrometer detects gaseous samples that have gained a proton, resulting in an M/Z value one higher than the relative molecular mass (M/R).
- For a substance with an M/Z of 113, the actual relative molecular mass is calculated as 113 minus 1, equating to 112, due to the added hydrogen ion.
- Chlorine molecules (Cl2) present a complex mass spectrum with potential different atomic masses for each chlorine atom, specifically 35 and 37.
- The possible mass combinations for Cl2 are 70 (35+35), 72 (35+37), and 74 (37+37), leading to three distinct peaks in the mass spectrum.
- The abundance ratios for chlorine isotopes are 9:6:1, with 75% of chlorine being Cl-35, resulting in the tallest peak at 70, followed by 72, and the smallest at 74.
- Key equations for mass spectrometry include speed (V = D/T) and kinetic energy (KE = 1/2 MV²), where V is in meters per second, D in meters, and T in seconds.
- The mass of one ion is derived from the relative atomic mass divided by 1000 and then divided by Avogadro's number (6.022 x 10²³) to convert grams to kilograms.
- A shortcut for calculating time of flight involves equating the relative atomic mass of isotopes to their time of flight squared, simplifying calculations significantly.
- The average relative atomic mass of chlorine is calculated by multiplying the abundance of each isotope by its mass, summing these, and dividing by total abundance, yielding 35.5.
- When given an average mass, such as 55.8 for iron, a number line can be used to visualize and calculate the contributions of different isotopes, aiding in understanding isotope distribution.
01:09:09
Isotope Abundance Calculation Techniques
- Divide the average atomic mass into ten chunks, marking 55.6, 55.5, and 55.4; 80% of iron is Fe-56, moving 8 out of 10 steps towards 56.
- For chlorine isotopes, split the range into four chunks; moving one quarter towards Cl-37 indicates 25% Cl-37 and three quarters towards Cl-35 indicates 75% Cl-35.
- To find magnesium isotopes' abundances, set the equation with X for Mg-24 and 90-X for Mg-25, knowing Mg-26 is 10% and the average is 24.3.
- Rearranging gives 24.3 multiplied by 100 equals 24X plus 90X, leading to X equaling 80%, indicating Mg-24 is 80%, Mg-25 is 10%, and Mg-26 is 10%.
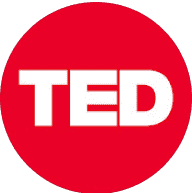
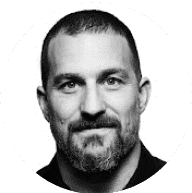
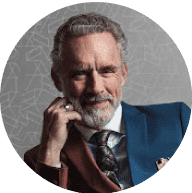

