U4.1: The Molecular Basis of inheritance
Wolfe Biology Lectures - ARC・5 minutes read
Unit four explores molecular genetics, highlighting DNA as the molecule of inheritance through various experiments and discoveries. Key concepts include DNA structure, replication, importance of base pairing, and mechanisms like proofreading and repair systems to maintain DNA integrity.
Insights
- DNA was identified as the molecule responsible for inheritance over proteins through experiments by Griffith, Hershey, and Chase, solidifying its role as the blueprint of life.
- Chargaff's research established the consistent ratios of A=T and G=C in DNA across organisms, reinforcing DNA's universal presence and importance in organismal diversity.
- DNA replication involves intricate processes like unwinding, strand synthesis, and proofreading, managed by enzymes like helicase, DNA polymerase, and DNA ligase, ensuring accurate copying and maintaining DNA integrity.
Get key ideas from YouTube videos. It’s free
Recent questions
What molecule is considered the blueprint of life?
DNA
Related videos
Summary
00:00
"DNA: Blueprint of Life and Inheritance"
- Unit four focuses on molecular genetics, transitioning from Mendel and Morgan's chromosome studies to DNA exploration.
- The primary focus is on DNA's discovery as the molecule of inheritance and the enzymatic process of DNA replication.
- Morgan's discovery highlighted that traits of inheritance were localized on chromosomes, leading to the debate between DNA and protein as the molecule of inheritance.
- Frederick Griffith's experiments with Streptococcus pneumoniae revealed the lethal S strain with a polysaccharide capsule and the non-lethal R strain, showcasing the impact of the capsule on white blood cell recognition.
- Griffith's transformation experiments demonstrated that DNA, not protein, was responsible for the transformation of R bacteria into S bacteria.
- Hershey and Chase's bacteriophage experiments confirmed DNA as the genetic material, using radioactive isotopes to track DNA's role in infection.
- DNA was found to be the molecule directing the host cell to produce new viruses, solidifying its identity as the blueprint of life.
- The Hershey-Chase experiment revealed that DNA, not protein, was responsible for infection, with DNA entering the host cell while the protein remained outside.
- DNA structure includes a deoxyribose sugar, phosphate group, and one of the four nitrogenous bases (A, G, C, T), with polymers formed by dehydration synthesis.
- Chargaff's research confirmed that the percentages of A=T and G=C in DNA are consistent across all organisms, providing evidence of DNA's diversity and role in organismal diversity.
17:29
"DNA Discovery: From Mendel to Replication"
- Mendel's three-to-one ratios indicated a universal phenomenon, leading to Chargaff's similar idea.
- Watson, Crick, Franklin, and Wilkins utilized Chargaff's ratios of ATGC to construct the double helix model.
- The equal numbers of A&T and G&C in organisms are due to base pairing, crucial for DNA structure.
- Variations in base percentages among organisms suggest diversity and potential for differences.
- Rosalind Franklin's x-ray crystallography revealed DNA's helical shape, pivotal in DNA discovery.
- Watson and Crick's assimilation of data from various sources led to the double helix model.
- The DNA molecule's phosphodiester backbone remains consistent, with information stored in base sequences.
- The anti-parallel nature of DNA strands, with major and minor grooves, aids in protein interaction.
- Hydrogen bonds between bases facilitate DNA unzipping for replication, following base pairing rules.
- Messelson and Stahl's experiments confirmed DNA replication as semi-conservative, using radioisotopes to track DNA generations.
38:14
"DNA Replication: Timing, Machinery, and Phases"
- Extra neutrons are shifted to light nitrogen to allow replication, with timing determining the number of replications.
- E. coli replicates every 20 minutes, starting heavy and then transitioning to light DNA strands.
- Semi-conservative DNA replication involves heavy-labeled parental strands and subsequent light strands.
- Density centrifuge analysis confirms the semi-conservative model of DNA replication.
- DNA replication involves separating DNA strands, assembling replication machinery, and following the ATGC rule.
- DNA replication requires parental strands, enzymes like DNA polymerase, and nucleotide triphosphates.
- Prokaryotic and eukaryotic DNA replication share initiation, elongation, and termination phases.
- DNA polymerase builds new DNA strands based on complementary base pairing and directionality.
- Prokaryotic replication starts at a specific origin sequence, forming replication bubbles for copying.
- Enzymes like helicase, primase, and DNA polymerase are crucial for unwinding and synthesizing new DNA strands.
56:07
Enzymes and processes in DNA replication
- Topoisomerase and DNA gyrase are enzymes involved in the physics of DNA replication, managing stress and preventing DNA breakage during unwinding.
- Helicase unwinds DNA, creating single-stranded regions, leading to the formation of coils upstream due to twisting.
- Gyrase cuts and repairs DNA strands to prevent tension buildup and potential breakage, ensuring the helicase can continue its unwinding process.
- Lagging strand synthesis is more complex than leading strand synthesis due to the anti-parallel nature of DNA, requiring the creation of fragments that are later joined together.
- Primase initiates fragment formation on the lagging strand by creating RNA primers, followed by DNA polymerase adding complementary DNA nucleotides to complete the fragments.
- DNA ligase plays a crucial role in linking the okazaki fragments on the lagging strand to form a continuous DNA strand.
- Eukaryotic DNA replication is more intricate due to the larger amount of DNA, linear chromosomes, and the need for multiple origins of replication to efficiently copy the extensive DNA.
- Eukaryotic replication follows a similar enzymatic process as prokaryotic replication, with additional enzymes involved in managing the challenges posed by linear chromosomes.
- Multiple origins of replication in eukaryotes allow for efficient copying of the larger chromosomes, with replication rates varying based on developmental stages and tissue requirements.
- Linear chromosomes in eukaryotes lead to the formation of multiple replication bubbles, with replication forks eventually merging to complete the replication process.
01:13:21
"Telomeres and DNA Replication: Safeguarding Genetic Material"
- DNA replication is akin to copying a book, where multiple people can copy pages simultaneously to expedite the process.
- To enhance DNA copying speed, initiating replication at multiple points simultaneously is crucial.
- Chromosome ends pose a challenge as polymerase one cannot copy them, leading to shortening over time.
- Telomeres, non-coding DNA sequences at chromosome tips, prevent the loss of vital genetic material during replication.
- Telomerase, an enzyme, extends telomeres by adding repeating bases, safeguarding important DNA during replication.
- Telomerase activity varies, being active in early cell stages but inactive in most somatic cells, affecting cell lifespan.
- Telomerase activation in cancer cells contributes to their indefinite division, offering insights into cell immortality.
- DNA replication is highly precise, with mechanisms like proofreading by DNA polymerase and repair systems for errors and damage.
- Mismatch repair and excision repair are vital processes to rectify base pairing errors and damaged DNA regions.
- Enzymes like photolyase can address issues like thymine dimers caused by UV light, ensuring DNA integrity.
01:30:46
DNA Repair, Mutations, and Evolutionary Adaptation
- Despite efforts to repair damaged DNA, mutations leading to conditions like cancer can still occur.
- Over evolutionary periods, the significant time allows for mutations to become more prevalent.
- The focus for the upcoming semester will be on DNA replication, transcription to RNA, and translation processes.
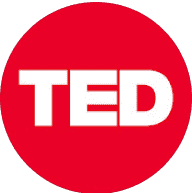
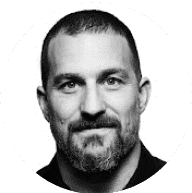
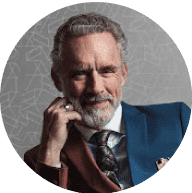

